Chapter 3: The 27 million year cometary cycle
Until the 1980’s cometary impacts as the main cause of mass extinctions was still controversial. Despite the understandable[1] scarcity of dated impact craters, the cometary induced mass extinction scenario gained a lot of traction with the discovery of a very distinct cycle punctuating most mass extinctions experienced by our planet. But before addressing this point and this mounting evidence of the cometary impacts, let’s have look at our solar system.
As everybody knows, our solar system is powered by a single star, the Sun. Well, it is assumed that ours is a single-star solar system because we see only one sun rise each morning. However, this is actually quite a peculiar configuration, since most stars astronomers have observed are part of multi-star systems (most often binary).
Based on data from NASA’s Chandra X-ray observatory, it’s estimated that over 80% of all stars may be in either binary or multiple-star systems.[2] Grazia and Milton, who studied the 60 star systems nearest to our own reached a comparable conclusion:
61% of the 60 nearest stars are components of a double (binary) or triple star system.[3]
A twin-star model for our own solar system is a tantalizing prospect, not least because it could account for many ‘anomalies’ exhibited by the single-star hypothesis. As stated by the Binary Research Institute (BRI):
elliptical orbit equations have been found to be a better predictor of precession rates than Newcomb’s formula, showing far greater accuracy over the last hundred years. Moreover, a moving solar system model appears to solve a number of solar system formation theory problems including the sun’s lack of angular momentum. For these reasons, BRI has concluded our sun is most likely part of a long cycle binary system.[4]
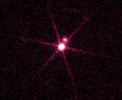
© NASA
Sirius is a binary star. Sirius A is the brightest.
Sirius B is dimmer and so close to Sirius A that it was not sighted until 1862.
Bear in mind that the binary systems identified above are composed of stars bright enough to be detected with a telescope. This means that the percentage of binary systems may be even higher, since some systems can include ‘unlit’ stars, like so-called ‘brown dwarfs’, for example.
For plasma cosmologists, a binary system is the logical way for individual stars to cope with high electric stress, causing any given star to go through a process of fission (i.e. splitting into two or more parts).[5] When a sphere is divided into two equally-sized spheres, the total mass will remain the same (no matter disappears) but the total surface area of this pair will be about 26% larger than the area of the original single sphere.[6] This increases the total surface area exposed to the electric field and thus decreases the current density (amperes per square meter). Thus, electrically-induced fission enables stars to reduce the electric stress they are subjected to by spreading it between two or more stars.
Because of the lower level of electric stress exerted on a binary system after fission, brown dwarfs (stars exposed to a weak electric field, hence their reduced brightness) should be quite common in binary systems:
It’s clear that binary stars are very common, probably even more common than acknowledged in the scientific literature. So, is our Sun one more anomaly in the rather anomalous universe depicted by mainstream science? Is our Sun really single?If the members of a resulting binary pair turn out to be unequal in size, the larger one will probably have the larger current density – but still lower than the original value. (This assumes that the total charge and total driving current to the original star distributes itself onto the new stars proportionally to their masses.) In this case, the smaller member of the pair might have such a low value of current density as to drop it, abruptly, to ‘brown dwarf’ or even ‘giant gas planet’ status.[7]
A significant clue that our star may in fact be part of a binary system appeared in Nature in the year 1982,[8] when paleontologists David Raup and Jack Sepkoski unveiled a cyclical pattern of mass-extinction events in the fossil record.[9] Their research revealed that over the last 250 million years, the Earth regularly experienced mass extinctions, as depicted in the chart below:
In the graph above we can see, out of 19 events - circled points-, 11 - green circled points - lie on the vertical lines showing the 27-My (million year) interval.
The above strongly suggests that mass extinctions, at least a majority of them, are due to a cyclical phenomenon, but doesn’t clarify the nature of this phenomenon.
The mystery lasted until Berkeley physicist Richard Muller discovered another significant clue 1986 when he compiled the iridium levels measured at the K/T boundary, which marked the mass extinction that occurred about 66 million years ago and matches the 27 My-cycle (3 cycles ago).
Muller checked the iridium levels in no less than 66 different locations across the whole planet. As shown on the map below, the results were unexpected: in each of those 66 different locations, Muller found abnormal levels of iridium.[10]
The discovery of Muller was confirmed by several researchers amongst which Frank T. Kyte and John T. Wasson who measured iridium in 149 samples of Pacific abyssal clay covering the time span 33 to 67 million years ago. They showed a prominent spike at K/T boundary (66 Mya) with an increase 200-fold compared to the background concentration[11].
What’s the big deal with iridium, you might wonder. Together with gold, platinum, osmium and rhenium, iridium is one of the ‘noble elements’ which are 10,000 times as abundant in meteoric material than in the Earth’s crust.[12] But it’s not just meteors that deliver these elements to Earth; the massive radiations emitted by supernovas can also generate large quantities of heavy elements, including iridium.
So, while iridium anomalies at the K/T boundary signal that the mass extinction about 66 million years ago was due to a cosmic event, it remained to be seen whether the culprit was a supernova or a meteor bombardment.
Initially, Luis Alvarez[13] was a proponent of the supernova theory. To prove his case he reasoned as follows: Pu-244, one of the isotopes of plutonium, isn’t naturally present in the Earth’s crust, nor in meteorites. However, it is one of the heavy elements created by supernovas. So if mass extinctions were due to supernova explosions, then we should find abnormally high levels of Pu-244 in the geological era boundary material because of its very long half-life of 80 million years. The analysis of Pu-244 concentrations in the clay sample from the E-O boundary led to the following conclusion:
There was no plutonium. A supernova had not killed the dinosaurs. These results were later published in a paper titled ‘Negative results for the Supernova hypotheses’. In this paper they [Alvarez et al.] described how the measurements showed there was no plutonium-244 present, and how this result ruled out the supernova theory[14]
If it was not a supernova, then the only possible cause was an asteroid / cometary bombardments (which we can refer to interchangeably[15]). Since then, several research teams, including University of Louisiana astronomer Dr. Daniel Whitmire and the Mellot and Bambach[16] team, have published papers confirming that meteor bombardments were indeed the cause of these cyclical mass extinction events.
Once it was established that cyclical mass extinctions were due to space rocks, another question arose: what is the main driver of these cyclical bombardments? The idea that a single asteroid or a swarm of asteroids can follow a stable 27-million-year orbit is highly unlikely.
Small celestial objects cannot remain in stable and very long orbits for hundreds of million years. As we’ve seen repeatedly in recent years, when comets approach massive celestial objects like Saturn, Jupiter or the Sun they are either destroyed, disintegrated, drastically weakened, ‘sucked in’ (hence the name ‘sun-diving comets’), or the encounter alters their orbit as they’re violently ejected back out of the inner solar system. So it is very unlikely that an asteroid swarm alone could cross the solar system, maintain a stable orbit throughout the journey, then return to the inner solar system on its next revolution and do it again and again for over 500 million years as suggested by Raupp and Sepkovsky’s research[17].
In addition such a long orbit implies that the hypothetical asteroid(s) would travel several light years away[18] from the Solar system and be subjected to the disruptive gravitational forces of local stars.
If asteroid(s) can’t follow such a stable 27-MY orbit by themselves, then something else obviously does; something large enough to maintain a stable orbit; and something that regularly sends fresh comets our way. That’s where Richard Muller came up with the idea that a solar companion – a twin Sun – followed such an orbit. Every 27 million years, on its approach towards the solar system, such a companion would disturb asteroids or comets local to its orbit, pushing and pulling them along its trajectory. Muller named this hypothetical Sun’s companion Nemesis[19] after the Greek goddess who relentlessly persecutes the excessively rich, proud and powerful.
After Muller’s ground-breaking work, several research teams, including astronomer Frank Low[20] and Thomas Chester[21], began exploring the sky to find Nemesis. This quest lasted for years but returned no positive results, so observers have yet to find still Nemesis. But as we noted earlier, some stars are not bright at all, as is the case with brown dwarfs (Dan Whitmire’s star category of choice for Nemesis[22]) and, to a lesser extent, extinct red dwarfs, which is Muller’s choice[23].
If the Sun’s companion is a brown dwarf – and if it is also orbiting in the ecliptic plane – finding it would be literally like finding a dark, invisible object surrounded by millions of bright, shining ones, a feat way more difficult than finding a needle in a haystack. This is quite an apt metaphor since up until now the technology hasn’t existed to allow us to successfully and systematically detect dark celestial bodies like brown dwarfs, as Whitmire explains:
Currently, I am searching the half billion point sources in the 2MASS database for evidence of this object. This survey covered 99% of the sky at near-infrared wavelengths of 1–2 microns. The optimum wavelength for our search is 5 microns but no such full sky survey exists, as yet.[24]
If Nemesis exists, the fact that it hasn’t been found despite years of research strongly advocates for it being a dark star.
As a side note, according to mainstream science, brown dwarfs are small stars nearing the end of their life. In standard cosmology, their ‘internal Fermi reactions’ are decreasing due to lack of fuel (hydrogen), making them progressively dimmer and dimmer. However, there are several problems with this model. For starters, brown dwarfs emit X-rays:
In standard astronomical models, Brown dwarfs are ‘supposed to be’ too cool and too small to maintain fusion reactions in their cores. The minimum temperature ‘should be’ three million degrees kelvin and the mass should be at least seven percent of the Sun’s mass, but some ‘brown dwarfs’ do not meet those requirements, they can’t trigger nuclear fusion although they emit X-ray radiation. [26]The orbiting x-ray telescope Chandra recently discovered a brown dwarf (spectral class M9) emitting an x-ray flare. This poses an additional problem for the advocates of the stellar fusion model. A star this cool should not be capable of x-ray flare production. How ‘gravitational collapse’ can produce x-rays remains unexplained.
‘We were shocked,’ said Dr. Robert Rutledge of the California Institute of Technology in Pasadena, the lead author on the discovery paper to appear in the July 20 issue of Astrophysical Journal Letters. ‘We didn’t expect to see flaring from such a lightweight object. This is really the ‘mouse that roared.’[25]
But a brown dwarf presents no anomaly in electric models. It’s simply a star that is not glowing because the local electric field is too weak. From this perspective it’s not the size (and therefore the limited gravitational field) that makes a star dark, but the electric stress. If the electric stress is too low, the star (whatever its size) doesn’t glow. Thus the size and temperature range determined by mainstream science to define brown dwarfs is irrelevant.
Now, let’s go back to the main idea of this chapter: the 27-My extinction cycle and its cometary nature.
In 1982[27], Raup and Sepkoski discovered that most major extinctions coincide with a 27 million years cycle. In 1980[28], Alvarez proved that the K/T extinction that wiped out the dinosaurs 66 Mya (3 cycles ago) was due to cometary bombardments. The combination of these two pieces of evidence strongly suggests that the 27 million year cycle was, at least partly, of a cometary nature, but that’s not all.
In 2017, almost 40 years after Alvarez, Raup and Sepkoski discoveries, New York University geologist and NASA consultant Michael Rampino[29] discovered that four of the six largest known impact craters of the last 250 My are correlated with three recognized extinction events, which coincide with the 27-million year cycle:
- the Chesapeake and Popigai impact craters ca. 34 Mya coeval late Eocene extinction (2 cycles ago) confirmed by anomalous level of iridium independently detected by Kyte[30] and Alvarez [31]
- the Chicxulub impact crater ca. 66 Mya (3 cycles ago) coincident with the end Cretaceous (or K/T) extinction
- the Morokweng/Mjolnir impact crater ca. 145 Mya (6 cycles ago), Jurassic-Cretaceous extinction
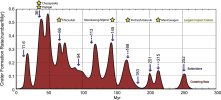
© Rampino
Correlation between the 6 largest known impact craters and five recognized extinction events
Notice also that, as indicated in the graph above:
- the end-Permian extinction ca. 252 Mya (10 cycles ago) coincidences with marked increase in impact crater formation rate although no time matching large impact crater has been identified yet.
- the Triassic-Jurassic extinction ca. 201 Mya (8 cycles ago) stratigraphic analysis reveals several evidence of cometary impact:
Diagnostic evidence of bolide impact in the stratigraphic record includes high-pressure (10–45 Gpa) mineral polymorphs, such as stishovite and coesite, and microscopic shock-deformation features in quartz, feldspar, and zircons[32]
In addition, three craters dating back to the times of this extinction (Manicouagan, Rochechouart and Saint Martin) are aligned[33] along the 22.8 N longitudes, which strongly suggest a crater chain formed by multiple successive impacts à la Shoemaker Levy.
At this point the correlation between the 27-My cycle, cometary bombardments and mass extinction is very strong.
We saw in the previous chapter that events like mass volcanism, ice ages or mass seismicity were proposed as the cause of several mass extinctions. We suggested that these events, while being a contributing factor to mass extinction, are the effects of cometary bombardments, which are, consequently, the primary triggering cause of extinctions.
This hypothesis was considerably strengthened in 2021 when Rampino[34] demonstrated that during last 260 My the last 89 major geological events, including cometary bombardments, mass volcanism, anoxia and sea level change and mass earthquakes where in sync with the 27-My cycle, as illustrated by the diagram below, which shows on the left the timing of major geological events (smoothed) while the graph on the right reveals the 27.5-My cyclicity of these major geological events as indicated by the black arrow:
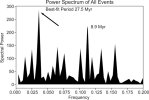
© Rampino
Timing (left) and cyclicity (right) of the 89 major geological events over the past 260 My
At this point, what is left to examine are extinction events outside the 27 My-cycle. Several of them also reveal the tell-tale signs of cometary encounters: impact craters and/or iridium and/or tektite:
- The mid-Ordovician extinction, also known as the Ordovician Meteor Event happened ca. 467 Mya. This extinction was marked by the dramatic increase in the rate at which chondrite meteorites[35] fell to Earth, by the Charlevoix Crater[36] and by a spike in iridium[37].
The Kellwasser Event (ca. 372 Mya) part the late Devonian extinction, is coeval with the Siljan[38] and the Alamo[39] crater, a concentration in iridium 20 times greater than the average[40] and a spike in micro-tektite[41].
The Hangelberg Event (ca. 358 Mya) part the late Devonian extinction, is synchronous with the Woodleigh crater[42]
- the Carboniferous-Permian extinction ca. 307 Mya also known as the Carboniferous rainforest collapse coeval with a iridium anomaly[43]
The above strongly suggests that mass extinctions, within the 27-My cycle and without, are, in a vast majority, caused by cometary impacts. What would happen to this proportion if the research in this field was not underfunded?
[1] Searching for impact craters on Earth is not an easy task because wind, rain, earthquakes, sea-level changes, vegetation growth and urbanization tend to erase these geological features. Plus, the search for impact craters doesn't seem to be a priority for modern science, maybe due to the strong ideological resistance – uniformitarianism - against the very idea of cometary impacts and their obvious catastrophic consequences. Nonetheless, at least three databases EDEIS , Somerikko and EID list some of the impact craters found on Earth. As of 2019, there are approximately 200 confirmed impact craters and 700 probable/possible ones despite the chronic under-funding this field of research.
[2] Cruttenden, W. (2005) “Lost Star”, St. Lynn's Press p.111
[3] De Grazia, A. & Milton, E.R. (2018), ‘’Solaria Binaria’’, Metron Publications p.17
[4] Binary Research Institute Editors (2011) “Introduction to Binary Companion Theory”, Binary Research Institute.
[5] Scott, D. (2006) “The Electric Sky”, Mikamar Publishing p. 157-159
[6] Scott, D. (2013) “Electric cosmology – Stellar Evolution”, Electric Cosmos.org
[7] Scott, (2006) p.158
[8] Raup, D. & Sepkoski, J. (1982), “Mass extinctions in the marine fossil record”, Science, 215- 4539, pp. 1501-1503
[9] According to calculations made by Raup & Sepkoski, the probability of a 27-MY mass extinction cycle being due to random chance is less than 1%.
[10] Muller, R. (1990) “Nemesis : The Death Star”, Arrow p.74-77
[11] Kyte FT, Wasson JT. (1986) “Accretion rate of extraterrestrial matter: iridium deposited 33 to 67 million years ago”. Science 232(4755):1225-9
[12] Muller, 1990 p.44
[13] At the time Luis Alvarez was the director of the Princeton research laboratories where Muller was conducting his research about Nemesis.
[14] Ibid., p.59
[15] Lescaudron, 2014. Chapter 18
[16] Melott A. & Bambach R., (2010) “Nemesis Reconsidered”, Monthly Notices of the Royal Astronomical Society Letters 407, issue 1, pp L99–L102
[17] Raup, D. & Sepkoski, J. (1982), “Mass extinctions in the marine fossil record”, Science, 215-4539, pp. 1501-1503
[18] An asteroid of neglectable mass (several tons) relative to the Sun would have an aphelion of roughly 200.000 AU that is 3.16 light years. Such a remote aphelion would subject the asteroid to the gravitational forces of nearby stars like Proxima Centauri (4.24 light years from the Sun) or the binary stars Alpha Centauri A and B (4.35 light years from the Sun) while the gravitational forces exerted by the Sun would be virtually null.
[19] Muller, 1990, p.114
[20] (1933-2009) Astronomer who worked for the University of Arizona
Jeff Harrison, (2009) “Astronomer Frank J. Low, 1933-2009” University of Arizona
[21] Thomas Jay Chester worked for NASA’s Jet Propulsion Laboratory (JPL)
Thomas Chester (2004) “Thomas Jay Chester's Website”
[22] D. Whitmire et al. (1984). ‘Are periodic mass extinctions driven by a distant solar companion?’, Nature 308, 713–715
[23] Muller, 1990 p.109
[24] Bruce A. (2009) “Science or Superstition”, Disinformation Books p.67
[25] Scott , 2006, p. 127
[26] Ibid, p. 127-129
[27] David M. Raup, J. John Sepkoski Jr. (1982). “Mass Extinctions in the Marine Fossil Record”. Science: 1501-1503
[28] Alvarez, L.W et al. (1980). "Extraterrestrial cause for the Cretaceous–Tertiary extinction". Science. 208 (4448):
[29] Rampino, Michael R.& Caldeira, Ken. (2017). “Correlation of the largest craters, stratigraphic impact signatures, and extinction events over the past 250 Myr”. Geoscience Frontiers, 8- 6, Pages 1241-1245,
[30] Kyte FT, Wasson JT. (1986) “Accretion rate of extraterrestrial matter: iridium deposited 33 to 67 million years ago”. Science 232(4755):1225-9
[31] Alvarez, W. et al. (1982) “Iridium anomaly approximately synchronous with terminal Eocene extinctions” Science, v. 216, p. 886-888.
[32] Tanner, Lawrence et al. (2004). “Assessing the record and causes of Late Triassic extinction”. Earth-Science Reviews. 65. 103-139. 10.1016
[33] Spray, J.G et al. (1998). “Evidence for a Late Triassic multiple impact event on Earth”. Nature 392,171–173
[34] Michael R. Rampino et al. (2021). “A pulse of the Earth: A 27.5-Myr underlying cycle in coordinated geological events over the last 260 Myr”. Geoscience Frontiers
[35] Korochantseva, Ekaterina et al. (2007). "L-chondrite asteroid breakup tied to Ordovician meteorite shower by multiple isochron 40 Ar- 39 Ar dating". Meteoritics & Planetary Science. 42 (1): 113–130.
[36] Schmieder, M. et al. (2019). “In situ U–Pb analysis of shocked zircon from the Charlevoix impact structure, Québec, Canada”. Meteoritics & Planetary Science. 54(8) pp. 1808-1827.
[37] Richard Grieve (1997) “Extraterrestrial impact events: the record in the rocks and the stratigraphic column” Palaeogeography, Palaeoclimatology, Palaeoecology
[38] Victor Clube, Bill Napier (1982) “The Cosmic Serpent: A Catastrophist View of Earth History” Universe Books p.121
[39] Sandberg, Charles et al. (2002). “Late Devonian sea-level changes, catastrophic events, and mass extinctions”. Geological Soc Am. 356. 473–87
[40] Playford PE, et al. (1984) “Iridium anomaly in the upper devonian of the canning basin, Western australia”. Science. 226(4673):437-9
[41] Sandberg, 2002
[42] Reimold, W.U. et al. (2003), “Woodleigh impact structure, Australia: Shock petrography and geochemical studies”. Meteoritics & Planetary Science, 38: 1109-1130.
[43] Mizukami, Takuya et al. (2014). “A spike of woody plant biomarkers in the deep-sea iridium layer at the Cretaceous/Paleogene boundary”. Palaeogeography, Palaeoclimatology, Palaeoecology. 412. 241–248.
Attachments
Last edited: