Part III: Viruses are the Drivers of Life
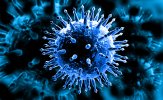
© Adobe StockGraphic
Representation of an Influenza virus
Chapter 9: The Enigma of Speciation
We concluded the chapter titled “Other life explosion” with the following statement: the above suggests that major cometary impacts are not only destructive acts through the removal of obsolete lifeforms during mass extinctions but also creative acts through the introduction of more elaborate lifeforms.
But what is the mechanism beyond these sudden life explosions?
Surprisingly, for a long time, this peculiarity didn’t attract much attention from the scientific community:
[…] the extinction of the dinosaurs and many other groups of animals at the
Cretaceous-Tertiary (K-T) boundary was the last of the six major mass extinction episodes identified around 1960 from the fossil record, the earlier ones (in chronological order) being near or at the end of the Cambrian, Ordovician, Devonian, Permian and Triassic Periods. However, very few evolutionary biologists or palaeontologists (investigators of fossils)
saw any reason to think a special explanation was required for these events. By this time, the Modern Synthesis, blending traditional Darwinism with population genetics, had become the overwhelmingly-dominant evolutionary paradigm, and
it was believed that the entire course of life on Earth could be explained through the mechanism of adaptive mutation.
[1]
Darwinists tentatively explain the evolutionary leaps by the vacancy of ecological niches enabling the apparition of new
species. This theoretical process is called
adaptive radiation[2]. It claims that the release from competition induced by mass extinction allows the ensuing evolutionary leaps.
If reduced competition was indeed the driver of evolutionary leaps, the most severe the mass extinction was, the lower the competition became and, consequently, the greater the evolutionary leap should be.
But it is not the case at all:
[T]here is no apparent relationship between the magnitude of an extinction and its ecological or evolutionary impact.
[3]
Actually paleontologists’ data show that competition or lack thereof plays no role in the origination of new species or the extinction of existing ones:
The deep-sea record shows that
species originations and extinctions are individual events unrelated to other species in the same environment. Competitive replacement (a fitter species leaking out of a marginal environment and outcompeting an established species) is generally not observed.
[4]
One can leave a bacterium in any environment as long as one wants; competition or not, it won’t lead to a
trilobite. This point is further illustrated by the previously mentioned ridiculously low probability
[5] of the advent of even the simplest cell. Vacant ecological space can’t account for the sudden apparition of new and more complex
species, whom, at least in some cases, are devoid of any known ancestors. While competitive replacement might play a marginal role in some instances, it is not enough, there’s obviously something missing.
According to recent research, mass extinctions do enable the subsequent evolutionary leaps:
There is no denying the profound evolutionary impetus mass extinctions have provided to the history of life.
Mass extinctions create new evolutionary opportunities and redirect the course of evolution. [6]
But how can mass extinctions (an act of destruction) enable life explosion (an act of creation) if not through the Darwinian competitive replacement? According to English biochemist and evolutionist
Trevor Palmer, the answer lies in genetics:
[…] developments in genetics have suggested that
mass extinctions may do more than create vacant ecological space for the radiation of new species. It seems clear that stress can give rise to
hypermutations and also to
epigenetic changes, so it must be considered possible that a catastrophic mass extinction episode could give rise to a range of variants beyond what was likely to have occurred during normal times
[7]
What could be the cause of the above-mentioned “hypermutations” and “epigenetic changes” that lead to the apparition of new
species?
By the way, the evolutionary leaps observed after mass extinctions require not only “hypermutations” as stated in the above quote but
beneficial hypermutations enabling the sudden appearance of not only new but also
more complex, more organized lifeforms. What could cause these fundamental and beneficial changes?
The apparition of new and more complex species after cometary-induced mass extinctions is a recurring pattern. This emergence of new species is called “speciation”, a process that has been observed in real time, only
once in animals
[8]:
Australian rock wallabies.
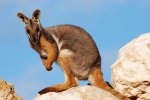
© Periptus
Yellow-footed rock wallaby (Petrogale xanthopus) at Monarto Zoo, Australia
Currently, the reorganizing of retroviruses in the genomes of is leading to the birth of a new species:
In 2001, O'Neill and her colleagues showed how retroviruses inhabiting the centromeres of the chromosomes of hybrid Australian rock wallabies are creating new species by wholesale juggling of chromosomal fragments.
[9]
This viral speciation hypothesis exposed in the above quote fits with
Trevor Palmer’s thesis according to which speciation is driven by “hypermutation” and “epigenetics changes”. Indeed, viruses are known to play a major role in epigenetics:
Viruses infecting animal cells are thought to play central roles in shaping the epigenetic scenario of infected cells. In this context it has become obvious that knowing the impact that viral infections have on the epigenetic control of their host cells will certainly lead to a better understanding of the interplay viruses have with animal cells.
[10]
Likewise, viruses are a major cause of “hypermutations” in hosts’ genomes through at least three processes:
1/ The integration of the viral genome in the host’s genome, a phenomenon also known as viral genome integration. This integration is a necessary step
[11] for all retroviruses and it occurs also for other viruses, including some as common as hepatitis B
[12], human herpes
[13] (HHV-6) or Epstein Barr
[14] viruses.
Let’s illustrate the viral genome integration with an example. A given animal is exposed to a new virus, carried by air, water or other lifeforms. At this point in time, for this animal, the virus is an exogenous virus. If the viral infection and integration occurs in the germline of this host, the virus sequence will become part of its progeny’s DNA, the exogenous virus has become an endogenous virus, and from now on it is a hereditary viral sequence integrated in the host descendants’ genomes. This integration of viral genetic sequences into a host’s DNA modifies it de facto as shown in the following illustration:
© Yoder Lab
viral integration into host genome
2/ once the virus is integrated,
it can further alter the host’s genome through a number of modifications amongst which duplication
[15], deletion
[16], replication
[17] and recombination hotspots
[18].
3/ viral sequences inserted in a host’s genome modify the expression of this genome, in other terms they turn on and off the existing genes
[19] of the host’s genome. This point will be developed in the next pages.
Actually,
the virus-induced modifications of the host’s genome are so deep that it can lead to fundamentally opposite results: death on one hand, evolution on the other one, depending on the host. This duality is reminiscent of recurring mass extinctions scenarios where the obsolete species face death while the sparred ones evolve:
Upon cell infection, some viruses integrate their genome into the host chromosome, either as part of their life cycle (such as retroviruses), or incidentally. While possibly promoting long-term persistence of the virus into the cell, viral genome integration may also lead to drastic consequences for the host cell, including gene disruption, insertional mutagenesis and cell death, as well as contributing to species evolution.
[20]
The ongoing speciation among Wallaby is not the only case of apparition of new lifeforms induced by viral sequences. The very apparition of the
genus homo (humans) is also associated with viral activity:
Humans share about 99% of their genomic DNA with chimpanzees and bonobos; thus, the differences between these
species are unlikely to be in gene content but could be caused by inherited changes in regulatory systems. Endogenous retroviruses (ERVs) comprise ∼ 5% of the human genome. The LTRs of ERVs contain many regulatory sequences, such as promoters, enhancers, polyadenylation signals and factor-binding sites. Thus, they can influence the expression of nearby human genes. […] It is likely that some of these ERVs could have integrated into regulatory regions of the human genome, and therefore could have had an impact on the expression of adjacent genes, which have consequently contributed to human evolution
[21]
In the quote above, the author makes a distinction between coding genes (i.e. that create proteins) and non-coding genes - that don’t create protein but instead regulate the activity of the coding regions.
To use an analogy, coding genes are like musicians who, instead of producing sound, produce proteins, which are the fundamental building-blocks of lifeforms. Non-coding genes play an even more fundamental role; they are like an orchestra director who, instead of directing musicians, directs
[22] the expression of coding genes.
© Jeffrey Zheng
Non-coding regions vs coding regions
As shown in the illustration above, coincidentally or not, most viral sequences are integrated
[23] in our non-coding genes, while they are very rare in coding regions
[24].
Now that we know a bit more about coding and non-coding regions, let’s go back to the apparition of humans and clarify the reason why viruses are the probable cause of the divergence between humans and other hominids (great apes).
- The genome difference between human and chimpanzees is only 0.5% in active coding regions
[25]. So the morphologic (also known as phenotypic) differences must come from non-coding regions.
- In the non-coding regions (the orchestra directors), the most active
[26] DNA sequences are called LTR (Long Terminal Repeat) and they are of viral origin
[27].
- Out 19 LTRs presence tested in human and other great apes genomes, 17 (~90%) were human specific.
[28]
The three points developed above show that the main genetic difference between humans and chimpanzees lies in viral LTRs, which are therefore the probable genetic cause of the obvious
morphologic differences.
Notice that the influence of viruses on the human genome and its expression didn’t stop with the divergence between humans from other great apes. Since then, viruses have been markedly shaping human DNA:
[…] an astonishing 30 percent of all protein adaptations since humans' divergence with chimpanzees have been driven by viruses.
[29]
Not only viruses are considered as the cause of the apparition of humans (genus homo) but the apparition of the whole taxonomic family (hominids) - to which they belong along with seven other extant species of great apes
[30] - is also attributed to viruses:
Hughes and Coffin used phylogenetic and sequence analysis to suggest that human endogenous retroviruses may have induced large-scale deletions, duplications and chromosome reshuffling in human genomic evolution. In the opinion of the geneticist Eugene Sverdlov, these viruses played a significant role in the evolution and divergence of the hominids.
[31]
One of the evidence provided by Sverdlov to correlate the hominids divergence with retrovirus activity is the conspicuous apparition of retroviruses in hosts’ genomes at the time great apes (hominids) diverged from other apes:
Some HERVs emerged in the genome over 30 MY ago, while others have appeared rather recently, at about the time of
hominid and
ape lineages divergence.
[32] [33]
The apparition of
placental mammals to which the
hominids family belongs is also correlated to the genomic integration of viral sequences
[34].
Coincidently or not, the earliest fossil of a
placental mammal dates back to 66 Mya, right at the time of the
Cretaceous–Paleogene extinction event. The fossil belongs to the
species Protungulatum donnae[35].
The taxonomic subclass constituted by
placental mammals is by far the most represented of the three subclasses of
mammals with nearly 4,000 species
[36] offering a broad morphologic diversity from the bat to the whale.
Until the advent of the
placentals, reproductive strategies were based on egg-laying.
Placentals brought a number of major innovations: placenta and uterus of course, but also the deactivation of the mother’s immune system against the fetus and the protection against infections of the fetus, which is virtually devoid of any immunity. ERVs play a major role in these innovations:
- They are necessary to the morphogenesis of the placenta
[37].
- They protect the fetus from infections by related exogenous retroviruses
[38]
- They protect the fetus from the mother’s immune system
[39]
- They control the expression of the embryo’s genome during its development
[40]
The illustration below shows the role played by some ERVs at each step of development from germ cell to fully formed embryo. Keep in mind that not all ERVs in placentals have been identified yet and even less ERVs have been characterized. Despite these limitations, the role played by ERVs is already omnipresent:
© Yangquan Xiang
Selective activation of some ERVs during host development
The
placental mammals (
eutherian) taxonomic subclass belongs to the
vertebrate taxonomic subgroup which appeared during the previously described
Cambrian explosion
[41] and coincides both phylogenetically
[42] and temporally with the apparition of retroviruses together with their vertebrate hosts:
[…] recent studies which date the emergence of the complex retroviruses of vertebrate lines at or just before the Cambrian Explosion of ∼500 Ma. Such viruses are known to be plausibly associated with major evolutionary genomic processes.
[43]
If we go further back the phylogenetic tree leading to
humans, we discover again the prominent role played by ERVs, this time in the creation of cellular nucleus
[44] and the apparition of one of the three
[45] domains of life, the
eukaryotes[46] (organisms whose cells have nucleus): which encompasses virtually all macroscopic lifeforms, including the previously described
vertebrates.
To recap, the apparition of our
genus (homo), the apparition of the
family it belongs to (
hominids), the apparition of the
subclass it belongs to (
eutheria), the
subphylum that contains it (
vertebrates) and the domain it belongs to (
eukaryotes) are each closely associated with the integration of ERVs in the hosts’ genomes.
In the diagram below, from left to right, the light grey arrow shows the apparition of the
eukaryotes, the white arrow shows the divergence of the
vertebrates from other
chordates[47], the medium grey arrow indicates the
eutheria divergence, the dark grey arrow shows the
great apes (hominids) divergence and the black arrow marks the
human (homo) divergence:
©Sott.net
Human phylogenic tree
To illustrate further the fundamental role played by viruses in speciation, the example of syncytin is edifying. Syncytin is a protein coded by a human endo-retrovirus called HERW1, involved in the specific development of
placental mammals described previously. The role of Syncytin is so cardinal that it is necessary for placental development:
Genetic studies in mice have established that the proteins encoded by syncytin A (Syna) and Synb, which arose independently in the rodent lineage from different ERV copies, are both required for the formation of the bilayered syncytiotrophoblast of the murine placenta.
[48]
Now, the unexpected feature of syncytin is that it was acquired by
mammals at least seven times, during distinct integration of distinct viruses and each time this integration is correlated with the aftermath of a speciation event:
[…] syncytin acquisition from distinct viruses has occurred independently at least seven times, each event happening after the divergence of the mammalian orders in which they are found.
[49]
In addition, paleovirological analyses established that syncytin-1 that is found in human that is around 30 million years old
[50], coeval with the
end-Eocene extinction.
© commons.wikimedia
Structure of the syncytin protein
The paradox raised by virtually identical genomes leading to different morphology is not limited to the above mentioned example of
homo vs chimpanzees. Actually, when compared to one another, most lifeforms exhibit the same contradiction. It is a thorny issue for Darwinists, according to whom evolution proceeds through incremental genetic changes, which lead to incremental morphologic changes. It is obviously not the case:
Results of recent research in evolutionary developmental biology is that the diversity of body plans and morphology in organisms across many phyla are not necessarily reflected in diversity at the level of the sequences of genes, including those of the developmental genetic toolkit and other genes involved in development. Indeed, as John Gerhart and Marc Kirschner have noted, there is an apparent paradox: "where we most expect to find variation, we find conservation, a lack of change". So, if the observed morphological novelty between different clades does not come from changes in gene sequences (such as by mutation), where does it come from? Novelty may arise by mutation-driven changes in gene regulation.
[51]
As detailed in the
human-chimpanzee divergence and applicable to virtually any lifeforms, viral sequences can and do cause different expressions of virtually identical genomes
[52], which solves the paradox exposed in the quote above.
There are a sheer number
[53] of viruses integrated in various hosts’ genomes, but their integration is not systematically dated. Nonetheless the examination of the scientific literature reveals four dates for the integration of three different virus families into mammals’ genomes:
- the integration of
bornaviridae ca. 93 Mya
- the integration of
circoviridae ca. 68 Mya
- the integration of
filoviridae and
parvoviridae, which are both dated to 30 Mya:
© Holmes et al.
Dating of the integration of viruses in hosts’ genomes
Notice that each of these four dating almost perfectly match the timing of some of the most recent mass extinctions:
- The
end-Eocene extinction ca. 34 Mya (cometary cycle #2)
- the
Cretaceous–Tertiary extinction ca. 66 Mya (cometary cycle #3)
- the
Cenomanian-Turonian extinction ca. 93 Mya (cometary cycle #4)
Beside the apparition of new families of viruses seems to be also associated with time of comet-induced mass extinctions. It is for example the case of the viral family
baculoviruses that contains 85 species of viruses
[54] and mostly infect insects
[55].
Baculoviruses are thought to have appeared ca. 310 Mya
[56], which is the time of the
Carboniferous-Permian extinction.
In this chapter, we’ve observed three strong correlations:
1/ ERVs and new taxa: ERVs are associated with the apparition of whole new
taxa;
species like
humans or
wallabies,
family like
hominids, subphylum like
vertebrates and even the taxonomic
domain eukaryotes.
2/ ERVs and mass extinctions: The integration of new viral families (
bornaviridae, circoviridae, filoviridae and
baculoviridae) and new viruses (the viral gene of syncytin-1) in hosts’ genomes is associated with the time of mass extinctions.
3/ New taxa and mass extinctions: the apparition of a number of new taxa is repeatedly associated with mass extinctions as extensively described part II.
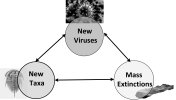
© Sott.net
Correlation between new taxa, new viruses and mass extinctions
Are these three associations fortuitous or do comet-induced mass extinctions mark the time when new ERVs are integrated in host genomes AND the time when these genomic integrations lead to the apparition of new lifeforms?
We have already seen two examples where these three associations are part of a single consistent chain of events:
a/ the divergence of the placentals, because of the genomic integration of a new virus during the
K/T extinction,
b/ the emergence of new viruses along with their vertebrate hosts during the
Cambrian explosion.
But the examples of the
placentals and the
vertebrates are not odd exceptions. Scientific literature reveals other instances when the integration of new ERVs was instrumental in the apparition of new species during or soon after a mass extinction:
- Jawed vertebrates appeared in the aftermath of the Ordovician–Silurian extinction. Analysis of their genomes reveals the viral origin of the RAG1 and RAG2 proteins and their pivotal role in the speciation of jawed vertebrates:
The results support the theory that RAG1 and RAG2 were once components of a transposable element [of viral origin
[57]], and that the split nature of immunoglobulin and T-cell-receptor genes derives from germline insertion of this element into an ancestral receptor gene soon after the evolutionary divergence of jawed and jawless vertebrates.
[58]
-
Teleost fishes appeared in the aftermath of the
Permian–Triassic extinction and their apparition was, at least partly, caused by viral sequences:
In contrast to mammalian genomes, teleost genomes also contain multiple families of active transposable elements [of viral origin
[59]], which might have played a role in speciation by affecting hybrid sterility and viability.
[60]
-
Lepidoptera: a whole order of insects containing 126 families
[61] and 180,000 species including moths, wasps and butterflies.
Lepidoptera represents 10% of the species of living organisms
[62] and appeared 200 Mya
[63] at the time of the
Permian-Triassic extinction without clearly identified ancestors
[64]. At the same time
[65] appeared a new family of viruses: the
bracovirus which lives symbiotically in virtually every
lepidopterian species[66].
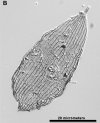
© Van Eldijk
Exquisitely fossilised Lepidoptera wing from the Triassic-Jurassic boundary
The information gathered above - in particular the apparition of the
vertebrates, the
teleost fishes, the
placentals the
jawed vertebrates and
Lepidoptera all occurring because the integration of new viral sequences around the times of mass extinctions - strongly suggest a causative chain of events involving the following time sequence:
comet-induced mass extinctions, apparition of new viruses, integration of viral sequences in hosts’ genomes and finally apparition of new taxa, as depicted in the diagram below:
© Sott.net
Comet → virus → speciation chain of event
[1] Palmer, Trevor (2018) “Perilous Planet Earth Revisited Chronology and Catastrophism” ResearchGate
[2] Givnish, T.J. (2015) “Adaptive radiation versus ‘radiation’ and ‘explosive diversification’: why conceptual distinctions are fundamental to understanding evolution” New Phytol, 207: 297-30
[3] Douglas H. Erwin (2001) “Lessons from the past: Biotic recoveries from mass extinctions”. PNAS, 98 (10) 5399-5403
[4] Cesare Emiliani (1994). “Evolution--a composite model” Evolutionary Theory, Vol.10, No.6, 299-303
[5] ~ 10-40,000
[6] Erwin, Douglas (2001) “Lessons from the past: Biotic recoveries from mass extinctions” PNAS 98 (10) 5399-5403
[7] Trevor Palmer (2010) “Lamarck – The Man, the Myth and the Legacy”. C&C Review, pp. 40-51
[8] Two other cases of real time speciation are often mentioned. The first if the Central European blackcap, a bird, which “could be on the verge of a speciation event”. The second is the Galapagos finch, whose genome analysis revealed that, after all, it was only a large cactus finch
See: Jennifer Skene (2010) “Evo in the news: Speciation in real time” UC museum of Paleontology
University of Berkeley contributors (2010) “Understanding Evolution: Speciation in real time” University of Berkeley
[9] Ryan, Frank. (2004). “Human endogenous retroviruses in health and disease: a symbiotic perspective”. Journal of the Royal Society of Medicine, 97(12), 560–565.
[10] Silvia C. Galvan et al. (2015).“Epigenetics and animal virus infections”. Editorial. Frontiers in Genetics.
[11] Desfarges, S., Ciuffi, A. (2012) “Viral Integration and Consequences on Host Gene Expression” Viruses: Essential Agents of Life, 147–175
[12] Murakami Y et al. (2005) “Large scaled analysis of hepatitis B virus (HBV) DNA integration in HBV related hepatocellular carcinomas” Gut 54:1162-1168
[13] Pellett PE et al (2011) “Chromosomally integrated human herpesvirus 6: questions and answers”. Rev Med Virol
[14] Gao J, et al. (2006) “Epstein-Barr virus integrates frequently into chromosome 4q, 2q, 1q and 7q of burkitt's lymphoma cell line” J. Virol. Methods 136:193-199
[15] Hughes JF, Coffin JM (2001) "Evidence for genomic rearrangements mediated by human endogenous retroviruses during primate evolution" Nature Genetics. 29 (4): 487–89
[16] Campbell, I. et al. (2014) “Human endogenous retroviral elements promote genome instability via non-allelic homologous recombination” BMC biology, 12, 74
[17] Ibid
[18] Mighel, A.J. et al (1997). “Alu sequences” FEBS Lettre 417, 1–5
[19] A gene is a sequence of DNA or RNA
[20] Desfarges, S. et al. (2012) “Viral Integration and Consequences on Host Gene Expression”. Viruses: Essential Agents of Life, 147–175
[21] Khodosevich, K. et al. (2002). “Endogenous retroviruses and human evolution.” Comparative and functional genomics, 3(6), 494–498
[22] Fernandes, J. et al. (2019) “Long Non-Coding RNAs in the Regulation of Gene Expression: Physiology and Disease”. Non-coding RNA, 5(1), 17
[23] De Parseval, N. et al. (2003) “Survey of human genes of retroviral origin: identification and transcriptome of the genes with coding capacity for complete envelope proteins” Journal of virology, 77(19), 10414–10422
[24] De Parseval, 2003
[25] Goodman M. (1999) “The genomic record of humankind’s evolutionary roots”. Am J Hum Genet 64: 31–39
[26] Boeke JD, Stoye JP. (1997). ‘’Retrotransposons, endogenous retroviruses, and the evolution of retroelements” In Retro- viruses, Cold Spring Harbor Laboratory Press 343 – 435
[27] Lower R, et al. (1996) “The viruses in all of us: characteristics and biological significance of human endogenous retrovirus sequences” PNAS 93:5177–5184
[28] Khodosevich, K. et al. (2002) “Endogenous retroviruses and human evolution.” Comparative and functional genomics, 3(6), 494–498
[29] Genetics Society of America (2016) “Viruses revealed to be a major driver of human evolution: Study tracking protein adaptation over millions of years yields insights relevant to fighting today's viruses” ScienceDaily
[30] 3 species of orangoutan, 2 species of gorilla, 1 species of chimpanzee and 1 species of bonobo. See:
Groves, C. P. (2005) “Mammal Species of the World: A Taxonomic and Geographic Reference (3rd ed.)” Johns Hopkins University Press. pp. 181–184
[31] Ryan, Frank (2004) “Human endogenous retroviruses in health and disease: a symbiotic perspective”. Journal of the Royal Society of Medicine, 97(12), 560–565
[32] Sverdlov ED. (2000) “Retroviruses and primate evolution” Bioessays;22(2):161-71
[33] Some of the ERVs that distinguish great apes from other apes are Fc2master and Fc2 env
[34] Chuong E. B. (2013) “Retroviruses facilitate the rapid evolution of the mammalian placenta” BioEssays : news and reviews in molecular, cellular and developmental biology, 35(10), 853–861
[35] O'Leary et al. (2013) "The Placental Mammal Ancestor and the Post–K-Pg Radiation of Placentals" Science. 339 (6120): 662–667
[36] Dave Smith (1994) “Eutheria, the Placental Mammals” University of Berkeley Museum of Paleontology
[37] Mi, S. (2000) “Syncytin is a captive retroviral envelope protein involved in human placental morphogenesis.” Nature 403:785–788
[38] Benit, L. (2001). “Identification, phylogeny, and evolution of retroviral elements based on their envelope genes.” J. Virol. 75:11709–11719
[39] Cianciolo, G. J. et al. (1985). “Inhibition of lymphocyte proliferation by a synthetic peptide homologous to retroviral envelope protein’’. Science 230:453–455
[40] Fu, B., et al. (2019). “Endogenous Retroviruses Function as Gene Expression Regulatory Elements During Mammalian Pre-implantation Embryo Development”. International journal of molecular sciences, 20(3), 790
[41] See Part II: Chapter “The Cambrian Life Explosion”
[42] Aiewsakun, P. et al. (2017) “Marine origin of retroviruses in the early Palaeozoic Era” Nature Communications 8, 13954
[43] Edward J. Steele, et al. (2018). “Cause of Cambrian Explosion - Terrestrial or Cosmic?” Progress in Biophysics and Molecular Biology, Volume 136, Pages 3-23
[44] Takemura M (2001) "Poxviruses and the origin of the eukaryotic nucleus". Journal of Molecular Evolution. 52 (5): 419–425
[45] The two other domains are the prokaryotes (bacteria) and the archaea (single-celled organism)
[46] Yoshikawa G et al. (2019) "Medusavirus, a Novel Large DNA Virus Discovered from Hot Spring Water" Journal of Virology. 93 (8)
[47] Taxonomic phylum including all lifeforms equipped with a backbone amongst other distinctive features
[48] Feschotte, C., Gilbert, C. (2012) “Endogenous viruses: insights into viral evolution and impact on host biology” Nature Review Genetics 13, 283–296
[49] Katzourakis A. (2013) “Paleovirology: inferring viral evolution from host genome sequence data” Philosophical transactions of the Royal Society of London. 368(1626), 20120493
[50] Lavialle, C., et al. (2013) “Paleovirology of 'syncytins', retroviral env genes exapted for a role in placentation” Philosophical transactions of the Royal Society of London. 368(1626), 20120507
[51] Wikipedia contributors (2021) “Evolutionary developmental biology” Wikipedia
[52] Rebollo R, et al. (2012) "Transposable elements: an abundant and natural source of regulatory sequences for host genes" Annual Review of Genetics 46 (1): 21–42
[53] See chapter : anteriority and pervasivness of Viruses
[54] Harrison, RL et al. (2018). "ICTV Virus Taxonomy Profile: Baculoviridae". The Journal of General Virology. 99 (9): 1185–1186
[55] Wang, M., & Hu, Z. (2019) “Cross-talking between baculoviruses and host insects towards a successful infection” Philosophical transactions of the Royal Society of London. 374(1767), 20180324
[56] Theze, J. et al. (2011) "Paleozoic origin of insect large dsDNA viruses". PNAS. 108 (38): 15931–5
[57] Young, G. R., et al. (2012) “Resurrection of endogenous retroviruses in antibody-deficient mice”. Nature, 491(7426), 774–778
[58] Agrawal, A., et al (1998) “Transposition mediated by RAG1 and RAG2 and its implications for the evolution of the immune system” Nature 394, 744–751
[59] Young, G. R. et al. (2012) “Resurrection of endogenous retroviruses in antibody-deficient mice” Nature, 491(7426), 774–778
[60] Volff, JN. (2005) “Genome evolution and biodiversity in teleost fish” Heredity 94, 280–294
[61] Capinera, John L. (2008) "Butterflies and moths" In “Encyclopedia of Entomology”. Springer. pp. 626–672
[62] Mallet, Jim (2007) "Taxonomy of Lepidoptera: the scale of the problem". The Lepidoptera Taxome Project
[63] van Eldijk et al. (2018) "A Triassic-Jurassic window into the evolution of Lepidoptera". Science Advances. 4 (1): e1701568
[64] J.-C. Sohn et al. (2015) “The fossil record and taphonomy of butterflies and moths (Insecta, Lepidoptera): Implications for evolutionary diversity and divergence-time estimates” BMC Evol. Biol. 15, 12
[65] Jennifer Welsh (2011) “Oldest Viruses Infected Insects 300 Million Years Ago” Live Science
[66] Gasmi L, et al. (2015) “Recurrent Domestication by Lepidoptera of Genes from Their Parasites Mediated by Bracoviruses” PLOS Genetics 11(9): e1005470