First, this post will briefly explain the process of cellular respiration. Then it will highlight the importance of proper mitochondrial function (bioenergetics) for maintaining the health of the organism, and will further show how poor mitochondrial function/energy production is an essential feature of nearly every known illness. When you understand the basics, then you can truly appreciate how Methylene Blue fits into the picture. So even though it will be long and tedious for some, it is highly recommended that you read all of the information before skipping to the studies at the bottom of the post.
How the cell produces energy: The basics of oxidative metabolism
According the current framework, the source of cellular energy is Adenosine Tri-Phosphate (ATP), which supposedly contains usable energy in its third phosphate bond. To make ATP, the body derives electrons and protons from food via digestion and subsequently breaks each molecule down into its constituent parts. The electrons and protons are passed along a series of molecules called redox (oxidation/reduction) molecules. Reduction refers to accepting electrons, and oxidation means to lose electrons. Redox molecules exist in two states, one being oxidised and the other being reduced.
In normal conditions, the (simplified) process is as follows:
Extracting energy from carbohydrate: 1. Glycolysis ===> 2. Acetyl CoA ===> 3. Krebs Cycle (TCA/Citric acid cycle) ===> 4. Electron transport chains/Oxidative Phosphorylation = ATP
Extracting energy from fat is slightly different: 1. Beta-Oxidation ===> 2. Acetyl CoA + Glycerol (which enters back into glycolysis) ===> 3. Krebs cycle (TCA/Citric acid cycle) ===> 4. Electron transport chain/Oxidative Phosphorylation = ATP
Below is a diagram of the first stage of glucose metabolism (glycolysis):
Notice the end product of this stage is Pyruvate (aka pyruvic acid) + 2 ATP + 2 NADH. This is a a redox reaction. NAD (Nicotinamide adenine dinucleotide) is a molecule derived from niacinamide (vitamin B3) and is a typical redox agent which participates in energy transfer. NAD+ is it's oxidised state, and NADH is it's reduced state. So in the above process, NAD+ is reduced (accepts electrons) so that it now becomes NADH. All that needs to be understood is that some of the electrons from food have been passed onto this carrier molecule (NAD), and that they will later to used to drive ATP synthesis in the final stage. However, to derive more of the energy from food, the next phase must proceed.
Under normal, healthy conditions in the presence of oxygen, most of the Pyruvate will be oxidised and will donate electrons to another molecule to produce Acetyl CoA. important note: pyruvate dehydrogenase is the enzyme which coverts pyruvate to Acetyl CoA.. This point is essentially the beginning of mitochondrial energy production, and aside from Acetyl CoA it also yields CO2 and more NADH.
The next stage involves Acetyl CoA entering the Krebs (citric acid) cycle whereby lots of redox reactions take place. This process yield more NADH, CO2, and FADH2. FAD (Flavin adenine dinucleotide) is derived from riboflavin (vitamin B2) and is similar to NAD - being a key redox component in ATP production. All that is important to know in this context is that more electrons are being derived from the original food product and are attached to carrier molecules to be used later. The Krebs cycle can be disrupted due to several factors, including if there is a deficiency of any of the intermediates involved, or a lack of oxygen.
For those who are wondering, this is now the point where fatty acids enter. Beta oxidation breaks down fatty acids into 2-carbon molecules and directly produces Acetyl CoA. So fatty acids basically bypass the whole process involving glycolysis and pyruvate oxidation.
Finally, the electron carriers NADH and FADH2 enter the "electron transport chain", which is made up of five protein complexes. The details on this can be found in biology text books, online, or on youtube for those who are interested.
Basically, NADH and FADH2 from each of the previous stages donate electrons the complex 1 and 2, which are passed to complex 3, and then finally through complex 4 to reduce oxygen and make water. This is the only stage in energy production where oxygen plays a role. But it is the final (and most important) stage, because when oxygen accepts those electrons it drives complex 5 (ATP Synthase) to produce 34 ATP. What is essential to understand here is that when there is no oxygen present, ATP cannot be synthesised in the mitochondria. .
RECAP
- Carbohydrate is broken down into glucose, which is converted into pyruvate via glycolysis. This yields 2 ATP.
- Pyruvate is converted into Acetyl CoA by the enzyme Pyruvate dehydrogenase when oxygen is present . In a similar way, fats are broken down into fatty acids, which also get converted into Acetyl CoA, and glycerol, which enters glycolysis.
-Acetyl CoA goes through the Krebs cycle to make NADH and FADH2
- Electrons from NADH and FADH2 run through the electron transport chain to reduce oxygen and this results in a massive production of ATP.
What happens when there is no oxygen?
When no oxygen in present, or when pyruvate dehydrogenase (the enzyme responsible for oxidising pyruvate into Acetyl CoA) is not functioning properly, the cell is left with only one option: glycolysis. Look back at the diagram of glycolysis. See that it only produces a measly 2 ATP, yet the only way it can do so is by having sufficient levels of NAD+ so that it can reduce them to NADH. In a situation where no oxygen is present, there are significant amounts of Pyruvate and NADH, and not enough NAD+ to keep the glycolytic cycle running.
In basic terms, the cell has a choice of 2 units of energy (ATP), or no energy at all (because there is a lack of oxygen). So in order to survive, it NEEDS to provide an avenue for NADH's electrons to be donated, otherwise energy production completely stops. Hence, it uses Pyruvate as the "electron sink", where NADH recycles back to NAD+ by donating it's electrons to pyruvate, which goes on to form lactic acid. The reaction is catalized by the enzyme lactate dehydrogenase. This is called anaerobic respiration, and is essentially a temporary measure made by the cell the survive in oxygen deprived conditions.
Main point: Energy is needed by every cell to function properly, to repair, and to differentiate into what is required at any given moment. Lactate and lactate dehydrogenase are markers of glycolysis - a highly inefficient energy source. This means that the level of lactate or lactate dehydrogenase enzymes can indicate whether the mitochondria are working properly so that there is enough energy to do what the cell needs to do, or whether it is resorting the the backup mechanism for whatever reason.
How does this look in real life?
Cancer
Type 1 and 2 Diabetes
Arthritis - RH and OA
Alzheimer's
Multiple Sclerosis
Consider that lactate dehydrogenase is just one biomarker that can be tested for. Looking through the research, it seems that mitochondrial inefficiency is a common factor in almost every pathology. Dr Doug Wallace, pioneering researcher on mitochondria, has essentially shown that mitochondrial heteroplasmy (mtDNA mutation rate) is associated with around 80% of diseases we see today. The following article briefly outlines some of his work:
Overwhelming evidence supports this notion, showing that defects in mitochondrial respiration underlie almost all illness.
The list is exhaustive, and anyone who is interested can do a search on PubMed and see that every non-genetic disease is essentially linked with mitochondrial respiratory defects. Ultimately, any factor which hampers the mitochondria's ability to produce enough energy is a potential disease-causing agent. A cell needs sufficient energy to be able to deal with life's stressors, and when that energy source declines, pathology kicks in. What this means is that energy metabolism is fundamentally coupled with a healthy living state. Nobel prize winner Albert Szent-Gyorgi emphasised in his book The Living State: With Observations on Cancer that "a living cell requires energy not only for all its functions, but also for the maintenance of its structure. He could see that structure and function were interdependent on every level, and he believed that in order for a system to increase its level of order and coherence, it must have sufficient energy to do so. Likewise, a lack of energy would decrease order, organisation, and would promote entropy in that system. This can be applied to the human body, and we see that the research supports this. The disease-state seems to be chaotic, where information transfer is poor and the system is incoherent as a whole, probably lacking in information. This is not limited to biological processes however, ala Gabor Mate and such individuals who show that the emotional/psychological state has the ability to promote or to cure disease. So in this sense, looking at the body almost like an information system which can be affected by all factors, external and internal, is probably the most constructive way to look at it. However, this thread is aimed at fixing some biological processes, so on this topic, I think we should attempt to increase the energy available to cell. We can do this by targeting the mitochondria, and by examining factors which are associated with defective mitochondrial metabolism.
Enter stress-metabolism
Any stressful stimuli (both external and physiological) requires increased metabolic efficiency to provide extra energy so that certain requirements can be met. If the stress persists, glycogen stores are eventually depleted (which happens fairly quickly), so adrenaline and cortisol are released via the HPA axis. Cortisol begins to break down muscle tissue, the liver performs gluconeogenesis and the proteins are converted to glucose. Adrenaline simultaneously activates lipolysis, breaking down adipose tissue and cell membranes fats to be used for energy by the mitochondria. However, due to the Randle Effect, fatty acids and glucose cannot undergo oxidative phosphorylation at the same time. Fatty acid oxidation inhibits pyruvate dehydrogenase (remember, the enzyme which allows pyruvate to enter the mitochondria), therefore glucose must undergo glycolysis. The simultaneous breakdown of fat via oxidative phosphorylation and glucose via glycolysis provides the body with maximum energy. However, the activation of HPA-axis hormones suppress the thyroid, and this process is only designed to be a temporary measure. The over production of lactate has several negative consequences, including further inhibition of mitochondrial respiration, and overall damage to cellular structures. A reliance on cortisol means that cholesterol's conversion to protective hormones pregnenelone, DHEA, and progesterone is stopped.
Ray Peat explains some of the processes involved in cancer "stress-metabolism" in the following:
The whole story is really complex, and there are so many avenues that link in with one another. I am only beginning to get my head around some of Peat's work, but I digress. A couple of things are for certain: Hypoxia (lack of oxygen) is present in almost every pathology, and in those that are not hypoxic, a defect in respiratory enzymes or complexes can be identified.
A small part of the picture: Nitric Oxide
Inefficient mitochondrial metabolism results in less production of carbon dioxide. Carbon dioxide is required by cells for several functional reasons, including the vasodilation of blood vessels, and maybe most importantly to allow the body to absorb oxygen via the respiratory tract. The amount of oxygen which can be absorbed from air is dependent on how much carbon dioxide is present in the blood, and this is due to the "Bohr Effect". Basically, if the mitochondria are not working properly, there will be low carbon dioxide (CO2), which also means that less oxygen diffuse into the blood stream. This is an initial cause of hypoxia. Remember that mitochondria need oxygen to produce ATP, so any oxygen deficiency is going to force many mitochondria to revert back to primitive glycolytic metabolism.
Additionally, since CO2 is the main vasodilator of blood vessels, in conditions of low CO2 the body is required upregulate the synthesis of Nitric Oxide (another potent vasodilator) via Nitric Oxide Synthase enzymes so that oxygen can reach the tissues. Nitric oxide elevations are meant to be a temporary measure against hypoxia.
However, during its own detoxification Nitric Oxide can bind "irreversibly" with Cytochrome C Oxidase, a key component of the mitochondrial electron transport chain. This means that for the cell to continue producing ATP via oxidative phosphorylation, it must produce a new cytochrome c oxidase, although this can be a lengthy and energy hungry process.
More on the destructive effects of excess Nitric Oxide:
This suggests to me that Nitric Oxide in any excess is likely to have a destructive effect on health, especially if the mitochondria are already working suboptimally.
Methylene Blue: A potential solution for restoring mitochondrial efficiency
Heres a chemical which has some pretty amazing properties, and can be used in many cases to increase mitochondrial function, and in some cases actually reverse pathology.
Among its many other benefits... I think some of the most interesting ones relate the it's effects on mitochondrial respiration:
1. Methylene Blue can artificially replace oxygen in the electron chain transport, meaning that hypoxia can essentially be mitigated and ATP synthesis can restored.
2. Methylene Blue can dissassociate Nitric Oxide from cytochrome C oxidase. From what I understand, the only other thing capable of doing this is red light.
3. Methylene Blue also inhibits Nitric Oxide Synthase
NOTE: It is best used in conjunction with red light, due to complementary physiological effects.
Here is an article from SelfHacked outlining some of the research on Methylene Blue:
How the cell produces energy: The basics of oxidative metabolism
According the current framework, the source of cellular energy is Adenosine Tri-Phosphate (ATP), which supposedly contains usable energy in its third phosphate bond. To make ATP, the body derives electrons and protons from food via digestion and subsequently breaks each molecule down into its constituent parts. The electrons and protons are passed along a series of molecules called redox (oxidation/reduction) molecules. Reduction refers to accepting electrons, and oxidation means to lose electrons. Redox molecules exist in two states, one being oxidised and the other being reduced.
In normal conditions, the (simplified) process is as follows:
Extracting energy from carbohydrate: 1. Glycolysis ===> 2. Acetyl CoA ===> 3. Krebs Cycle (TCA/Citric acid cycle) ===> 4. Electron transport chains/Oxidative Phosphorylation = ATP
Extracting energy from fat is slightly different: 1. Beta-Oxidation ===> 2. Acetyl CoA + Glycerol (which enters back into glycolysis) ===> 3. Krebs cycle (TCA/Citric acid cycle) ===> 4. Electron transport chain/Oxidative Phosphorylation = ATP
Below is a diagram of the first stage of glucose metabolism (glycolysis):
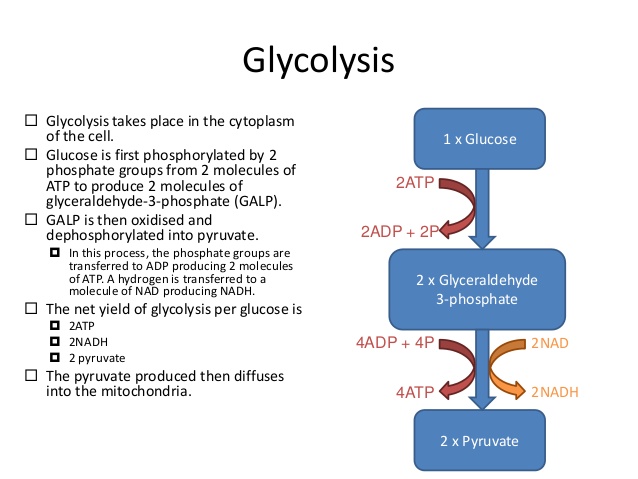
Notice the end product of this stage is Pyruvate (aka pyruvic acid) + 2 ATP + 2 NADH. This is a a redox reaction. NAD (Nicotinamide adenine dinucleotide) is a molecule derived from niacinamide (vitamin B3) and is a typical redox agent which participates in energy transfer. NAD+ is it's oxidised state, and NADH is it's reduced state. So in the above process, NAD+ is reduced (accepts electrons) so that it now becomes NADH. All that needs to be understood is that some of the electrons from food have been passed onto this carrier molecule (NAD), and that they will later to used to drive ATP synthesis in the final stage. However, to derive more of the energy from food, the next phase must proceed.
Under normal, healthy conditions in the presence of oxygen, most of the Pyruvate will be oxidised and will donate electrons to another molecule to produce Acetyl CoA. important note: pyruvate dehydrogenase is the enzyme which coverts pyruvate to Acetyl CoA.. This point is essentially the beginning of mitochondrial energy production, and aside from Acetyl CoA it also yields CO2 and more NADH.

The next stage involves Acetyl CoA entering the Krebs (citric acid) cycle whereby lots of redox reactions take place. This process yield more NADH, CO2, and FADH2. FAD (Flavin adenine dinucleotide) is derived from riboflavin (vitamin B2) and is similar to NAD - being a key redox component in ATP production. All that is important to know in this context is that more electrons are being derived from the original food product and are attached to carrier molecules to be used later. The Krebs cycle can be disrupted due to several factors, including if there is a deficiency of any of the intermediates involved, or a lack of oxygen.
For those who are wondering, this is now the point where fatty acids enter. Beta oxidation breaks down fatty acids into 2-carbon molecules and directly produces Acetyl CoA. So fatty acids basically bypass the whole process involving glycolysis and pyruvate oxidation.

Finally, the electron carriers NADH and FADH2 enter the "electron transport chain", which is made up of five protein complexes. The details on this can be found in biology text books, online, or on youtube for those who are interested.

Basically, NADH and FADH2 from each of the previous stages donate electrons the complex 1 and 2, which are passed to complex 3, and then finally through complex 4 to reduce oxygen and make water. This is the only stage in energy production where oxygen plays a role. But it is the final (and most important) stage, because when oxygen accepts those electrons it drives complex 5 (ATP Synthase) to produce 34 ATP. What is essential to understand here is that when there is no oxygen present, ATP cannot be synthesised in the mitochondria. .
RECAP
- Carbohydrate is broken down into glucose, which is converted into pyruvate via glycolysis. This yields 2 ATP.
- Pyruvate is converted into Acetyl CoA by the enzyme Pyruvate dehydrogenase when oxygen is present . In a similar way, fats are broken down into fatty acids, which also get converted into Acetyl CoA, and glycerol, which enters glycolysis.
-Acetyl CoA goes through the Krebs cycle to make NADH and FADH2
- Electrons from NADH and FADH2 run through the electron transport chain to reduce oxygen and this results in a massive production of ATP.
What happens when there is no oxygen?
When no oxygen in present, or when pyruvate dehydrogenase (the enzyme responsible for oxidising pyruvate into Acetyl CoA) is not functioning properly, the cell is left with only one option: glycolysis. Look back at the diagram of glycolysis. See that it only produces a measly 2 ATP, yet the only way it can do so is by having sufficient levels of NAD+ so that it can reduce them to NADH. In a situation where no oxygen is present, there are significant amounts of Pyruvate and NADH, and not enough NAD+ to keep the glycolytic cycle running.

In basic terms, the cell has a choice of 2 units of energy (ATP), or no energy at all (because there is a lack of oxygen). So in order to survive, it NEEDS to provide an avenue for NADH's electrons to be donated, otherwise energy production completely stops. Hence, it uses Pyruvate as the "electron sink", where NADH recycles back to NAD+ by donating it's electrons to pyruvate, which goes on to form lactic acid. The reaction is catalized by the enzyme lactate dehydrogenase. This is called anaerobic respiration, and is essentially a temporary measure made by the cell the survive in oxygen deprived conditions.
Main point: Energy is needed by every cell to function properly, to repair, and to differentiate into what is required at any given moment. Lactate and lactate dehydrogenase are markers of glycolysis - a highly inefficient energy source. This means that the level of lactate or lactate dehydrogenase enzymes can indicate whether the mitochondria are working properly so that there is enough energy to do what the cell needs to do, or whether it is resorting the the backup mechanism for whatever reason.
How does this look in real life?
Cancer
Serum lactate dehydrogenase and survival following cancer diagnosis.
RESULTS:
At the end of follow-up, 5799 participants were deceased. Hazard ratios (HRs) and 95% confidence intervals (CIs) for overall and cancer-specific death in the multivariable model were 1.43 (1.31-1.56) and 1.46 (1.32-1.61), respectively, for high compared with low prediagnostic LDH. Site-specific analysis showed high LDH to correlate with an increased risk of death from prostate, pulmonary, colorectal, gastro-oesophageal, gynaecological and haematological cancers. Serum LDH assessed within intervals closer to diagnosis was more strongly associated with overall and cancer-specific death.
CONCLUSIONS:
Our findings demonstrated an inverse association of baseline serum LDH with cancer-specific survival, corroborating its role in cancer progression.
https://www.ncbi.nlm.nih.gov/pubmed/26469834
Type 1 and 2 Diabetes
Lactate, a Neglected Factor for Diabetes and Cancer Interaction
Fasting plasma lactate level is increased in patients with DM including T1DM and T2DM versus nondiabetic persons [28–36]. Diabetic patients with obesity exhibit higher fasting plasma lactate levels than nondiabetic individuals with obesity [37, 38]. Barnett et al. proposed that diabetes-associated hyperlactatemia might be an early change in the time course of the disease [39]. Recently, Berhane et al. [40] demonstrated that lactate production progressively rises during hyperinsulinemic euglycemic clamp study, a condition of hyperinsulinemia similar to the early stages in the development of T2DM. Intriguingly, similar previous studies also report elevated lactate concentrations during the early stages of diabetes, prediabetes, and the hyperinsulinemia condition. In addition, Brouwers et al. [41] reported increased lactate levels in patients with poorly controlled T1DM and glycogenic hepatopathy, implying that enhanced plasma lactate concentrations are part of the clinical spectrum of these diseases. Furthermore, lactate has also been revealed to predict diabetes occurrence in the future [42, 43].
The mechanisms underlying diabetes-associated hyperlactatemia include serious changes in the intracellular glucose metabolism in insulin-sensitive tissues, for example, diminished glycogen synthesis, compromised glucose oxidative metabolism, and increased whole-body rate of nonoxidative glycolysis [28, 31, 44]. Importantly, when compared with controls, nonoxidative glycolysis rate retains higher in T2DM patients during hyperglycemic [31, 44, 45] and hyperinsulinemic [31, 44] status. In addition, the postprandially nonoxidative glycolysis is elevated in these patients relative to healthy controls and blood lactate level rises under this condition [36]. Insulin resistance plays a vital role in the pathogenesis of T2DM [46] and can be used as an early marker for the disease [40]. Under the insulin resistant condition, high levels of insulin promote glycolysis through activating two rate limiting enzymes, namely, phosphofructokinase and pyruvate dehydrogenase [47]. Thus, patients with insulin resistance/diabetes exhibit augmented activity of glycolysis [31, 48]. The elevated glycolysis results in enhanced formation of NADH and pyruvate and reduced NAD+ levels. Pyruvate is converted into lactate by LDH accompanied by NAD+ generation from NADH in a redox reaction. This reaction may be accentuated in insulin resistance since hyperinsulinemia induces enhanced glycolysis.
https://www.hindawi.com/journals/mi/2016/6456018/
Arthritis - RH and OA
Lactate dehydrogenase activity and its isoenzymes in serum and synovial fluid of patients with rheumatoid arthritis and osteoarthritis.
By determining the total activity of total lactate dehydrogenase (LDH-T) and its isoenzymes in serum and synovial fluid (SF) of patients with rheumatoid arthritis (RA) and osteo-arthritis (OA) we demonstrated in RA serum increased (p less than 0.02) activity of hepatic LDH (LDH-H) and a shift of the LDH isoenzymatic profile towards the M forms; in rheumatoid SF increased (p less than 0.001) activity of the total LDH-T and LDH-H which makes possible the use of these markers of inflammation in assessing RA activity. Values for LDH-T and LDH-H of 400-700 U/l and 300-500 U/l, respectively, correspond to moderate disease activity, while values exceeding 750 U/l and 550 U/l, respectively, correspond to high RA activity. The anaerobic isoenzymatic distribution of LDH in rheumatoid SF results in a significant (p less than 0.001) decrease in LDH1 and LDH2 and an increase (p less than 0.001) in LDH4 and LDH5
https://www.ncbi.nlm.nih.gov/pubmed/1593572
Alzheimer's
Activities of key glycolytic enzymes in the brains of patients with Alzheimer's disease.
The activities of hexokinase, aldolase, pyruvate kinase, lactate dehydrogenase and glucose 6-phosphate dehydrogenase were determined in brains of patients with Alzheimer's disease (AD) and in age matched controls. For pyruvate kinase and lactate dehydrogenase a significant increase in specific activity was found in frontal and temporal cortex of AD brains, while the activities of aldolase and hexokinase are not changed. Glucose 6-phosphate dehydrogenase activity was significantly reduced in hippocampus. The increase of some glycolytic enzyme activities is correlated with increased contents of lactate dehydrogenase and glial fibrillary acidic protein (GFAP) in homogenates of frontal and temporal cortex and elevated phosphofructokinase (PFK) and GFAP in astrocytes from the same brain areas. The data extend previous findings on an increase in brain PFK specific activity in AD and suggest that the increased activity of some glycolytic enzymes may be, at least in part, the result of the reactive astrocytosis developing in the course of AD.
https://www.ncbi.nlm.nih.gov/pubmed/10443553
Multiple Sclerosis
Lactic dehydrogenase isoenzymes in adolescents with multiple sclerosis.
Multiple sclerosis is an immune-mediated demyelinating disease with high morbidity and major mortality. To determine the potential use of lactic dehydrogenase activity and lactic dehydrogenase isoenzyme concentrations in cerebrospinal fluid as biomarkers of multiple sclerosis, we reviewed the files of all patients with multiple sclerosis who attended our tertiary pediatric medical facility from 1999-2005. The study group included three adolescent patients with multiple sclerosis (cerebrospinal fluid analysis at diagnosis) and one patient with recurrent optic neuritis (cerebrospinal fluid analysis during a disease episode). The isoenzyme pattern was abnormal in all patients with multiple sclerosis, with higher-than-normal levels of lactic dehydrogenase-2, lactic dehydrogenase-3, and lactic dehydrogenase-5 in two patients, and lower-than-normal levels of lactic dehydrogenase-4 in one patient. It was not necessarily, however, the same two patients who had the abnormally high levels of lactic dehydrogenase-2, -3, and -5. The patient with optic neuritis also exhibited an abnormal lactic dehydrogenase isoenzyme pattern that shared common features with the others. Multiple sclerosis appears to be characterized by an abnormal lactic dehydrogenase isoenzyme pattern in cerebrospinal fluid. The importance of this finding and its diagnostic potential use warrant further investigation.
https://www.ncbi.nlm.nih.gov/pubmed/19748045
Consider that lactate dehydrogenase is just one biomarker that can be tested for. Looking through the research, it seems that mitochondrial inefficiency is a common factor in almost every pathology. Dr Doug Wallace, pioneering researcher on mitochondria, has essentially shown that mitochondrial heteroplasmy (mtDNA mutation rate) is associated with around 80% of diseases we see today. The following article briefly outlines some of his work:
New work by a pioneering scientist details how subtle changes in mitochondrial function may cause a broad range of common metabolic and degenerative diseases. Mitochondria are tiny energy-producing structures within our cells that contain their own DNA.
The new research shows that small changes in the ratio of mutant to normal mitochondrial DNA within the thousands of mitochondrial DNAs inside each cell can cause abrupt changes in the expression of numerous genes within the nuclear DNA. Furthermore, the different proportions of mutant mitochondrial DNA that result in altered nuclear gene expression correspond to the same proportions of mutations in mitochondrial DNA that are associated with diabetes and autism; brain, heart and muscle disease; or lethal infantile disease.
“By showing that subtle changes in the cellular proportion of the same mitochondrial DNA mutation can result in a wide range of different clinical manifestations, these findings challenge the traditional model that a single mutation causes a single disease,” said study leader Douglas C. Wallace, Ph.D., director of the Center for Mitochondrial and Epigenomic Medicine at The Children’s Hospital of Philadelphia. He added, “The research offers key insights into understanding the underlying cause of metabolic and neurodegenerative disorders such as diabetes, Alzheimer, Parkinson and Huntington disease, as well as human aging.”
“The discrete changes in nuclear gene expression in response to small increases in mitochondrial DNA mutant level are analogous to the phase changes that result from adding heat to ice,” said Wallace. “As heat is added, the ice abruptly turns to water and with more heat, the water turns abruptly to steam.” Here a quantitative change (an increasing proportion of mitochondrial DNA mutation) results in a qualitative change (coordinate changes in nuclear gene expression together with discrete changes in clinical symptoms).
The study by Wallace and colleagues appeared online Sept. 3 in the Proceedings of the National Academy of Sciences.
Existing in hundreds or thousands of copies outside the nucleus of every cell, mitochondria have their own DNA, distinct from the well-known DNA inside the cell nucleus. Although mitochondrial DNA (mtDNA) holds far fewer genes than nuclear DNA, mtDNA exchanges signals with nuclear DNA and participates in complicated networks of biochemical reactions essential to life.
Wallace’s current study rests on his investigations into the mysteries of mitochondria for over 40 years. In 1988, he was the first to demonstrate that mitochondrial DNA mutations can cause human disease. He has continued to build a body of research into mechanisms by which mutations in mtDNA contribute to both rare and common diseases by disrupting the body’s energy production. In the current study, Wallace’s team investigated the impacts of steadily increasing levels of a pathogenic mutation in one particular base of mitochondrial DNA.
Researchers already knew that if 10 to 30 percent of a person’s mitochondrial DNA has this mutation, a person has diabetes, and sometimes autism. Individuals with an mtDNA mutation level of 50 to 90 percent have other multisystem diseases, particularly MELAS syndrome, a severe condition which involves brain and muscle impairments. Above the 90 percent level, patients die in infancy.
In the current study, conducted in cultured human cells, Wallace and colleagues analyzed cells with different levels of this pathogenic mtDNA mutation to determine the effects on the gene expression of the cell. The researchers measured variations in cellular structure and function, nuclear gene expression, and production of different proteins.
“The mutations in mitochondria impair their ability to produce energy, and mitochondria transmit distress signals to the cell nucleus,” said Wallace. “But the nucleus can respond in only a limited number of ways.” Those responses may manifest themselves in discrete, profound consequences for patients.
Findings May Pertain to Common Conditions
Wallace argues that the medical significance of this research extends beyond the province of the relatively rare disorders typically classified as mitochondrial diseases. The gene expression profile—the pattern of gene activity seen at the level at which mtDNA mutations trigger brain disorders—parallels the profiles found in Alzheimer, Parkinson and Huntington diseases. “The findings in this study provide strong support for the concept that common metabolic diseases such as diabetes and obesity, heart and muscle diseases, and neurodegenerative diseases have underpinnings in energy deficiencies from malfunctioning mitochondria,” said Wallace. “Thus this concept brings together a cluster of diseases previously considered to be separate from one another.”
Significantly, Wallace added that the research also pertains to aging. Because mitochondrial mutations accumulate as people age, mitochondrial energy production declines, with deleterious effects on the heart, the brain and on interrelated biological systems that sustain health and life.
Next steps in research, says Wallace, include investigations of how different diseases are associated with the sorts of abrupt phase changes his group found in the current cellular study. Some of the cellular changes, signaling patterns and protein activity levels found in the current research might become useful biomarkers in disease studies and drug development. “For instance, a preclinical screen for potential drugs that could reverse gene expression profile changes of the mitochondrial DNA mutant cells could reveal new therapies,” he added.
Wallace’s current study reinforces arguments he has presented over the course of his career, that mitochondria play a central, largely under-recognized role in all common human diseases. He has long argued that a traditional biomedical approach focusing on anatomy and individual organs does not provide the insights generated from a systems biology, bioenergetics-focused approach.
Wallace’s paradigm-shifting hypotheses remain controversial in biomedicine. This latest study, he says, implies that the complexity of common diseases is rooted in the disconnect between continuous, linear changes in mtDNA mutations and the discontinuous, sudden phase changes in nuclear gene expression that result. Even as his overall arguments about the role of mitochondria contend for broader acceptance, the current findings may provide useful, versatile tools for understanding and treating disease.
Overwhelming evidence supports this notion, showing that defects in mitochondrial respiration underlie almost all illness.
Neurodegenerative diseases
Mitochondrial defects are found in pathological studies of all major neurodegenerative diseases, said Vamsi Mootha of Harvard Medical School. The range of mitochondrial defects includes fragmentation and other morphological changes, increased mutation rates in mtDNA, changes in permeability of mitochondrial membranes, changes in redox potential, accumulation of mutant proteins, and impaired oxidative phosphorylation (Reddy and Reddy, 2011).
https://www.ncbi.nlm.nih.gov/books/NBK208519/
Cancer
As an alternative to the somatic mutation theory, emerging evidence suggests that cancer is primarily a mitochondrial metabolic disease (Seyfried and Shelton, 2010; Hu et al., 2012; Verschoor et al., 2013; Seyfried et al., 2014). The view of cancer as a metabolic disease originated with the experiments of Otto Warburg (Warburg, 1956a,b; Burk et al., 1967). Respiratory insufficiency is the origin of cancer according to Warburg's theory. All other phenotypes of the disease, including the somatic mutations, arise either directly or indirectly from insufficient respiration (Warburg, 1956a; Seyfried, 2012a; Seyfried et al., 2014). Warburg's metabolic theory was also in line with the concepts of C. D. Darlington and others showing that cancer is largely a cytoplasmic mitochondrial disease (Woods and Du Buy, 1945; Darlington, 1948).
[..]
In summary, the information presented here supports the notion that cancer originates from damage to the mitochondria in the cytoplasm rather than from damage to the genome in the nucleus. The genomic damage in tumor cells follows, rather than precedes, the disturbances in cellular respiration. This view is also consistent with the previous findings of Roskelley et al. (1943), Hu et al. (2012).
http://journal.frontiersin.org/article/10.3389/fcell.2015.00043/full
Skin diseases
Aberrant mitochondrial structure and function influence tissue homeostasis and thereby contribute to multiple human disorders and ageing. Ten per cent of patients with primary mitochondrial disorders present skin manifestations that can be categorized into hair abnormalities, rashes, pigmentation abnormalities and acrocyanosis. Less attention has been paid to the fact that several disorders of the skin are linked to alterations of mitochondrial energy metabolism. This review article summarizes the contribution of mitochondrial pathology to both common and rare skin diseases. We explore the intriguing observation that a wide array of skin disorders presents with primary or secondary mitochondrial pathology and that a variety of molecular defects can cause dysfunctional mitochondria. Among them are mutations in mitochondrial- and nuclear DNA-encoded subunits and assembly factors of oxidative phosphorylation (OXPHOS) complexes; mutations in intermediate filament proteins involved in linking, moving and shaping of mitochondria; and disorders of mitochondrial DNA metabolism, fatty acid metabolism and heme synthesis. Thus, we assume that mitochondrial involvement is the rule rather than the exception in skin diseases. We conclude the article by discussing how improving mitochondrial function can be beneficial for aged skin and can be used as an adjunct therapy for certain skin disorders. Consideration of mitochondrial energy metabolism in the skin creates a new perspective for both dermatologists and experts in metabolic disease.
https://www.ncbi.nlm.nih.gov/pubmed/24980550
Cardiovascular disease
Whereas the pathogenesis of atherosclerosis has been intensively studied and described, the underlying events that initiate cardiovascular disease are not yet fully understood. A substantial number of studies suggest that altered levels of oxidative and nitrosoxidative stress within the cardiovascular environment are essential in the development of cardiovascular disease; however, the impact of such changes on the subcellular or organellar components and their functions that are relevant to cardiovascular disease inception are less understood. In this regard, studies are beginning to show that mitochondria not only appear susceptible to damage mediated by increased oxidative and nitrosoxidative stress, but also play significant roles in the regulation of cardiovascular cell function. In addition, accumulating evidence suggests that a common theme among cardiovascular disease development and cardiovascular disease risk factors is increased mitochondrial damage and dysfunction.
https://www.ncbi.nlm.nih.gov/pubmed/15855047
The list is exhaustive, and anyone who is interested can do a search on PubMed and see that every non-genetic disease is essentially linked with mitochondrial respiratory defects. Ultimately, any factor which hampers the mitochondria's ability to produce enough energy is a potential disease-causing agent. A cell needs sufficient energy to be able to deal with life's stressors, and when that energy source declines, pathology kicks in. What this means is that energy metabolism is fundamentally coupled with a healthy living state. Nobel prize winner Albert Szent-Gyorgi emphasised in his book The Living State: With Observations on Cancer that "a living cell requires energy not only for all its functions, but also for the maintenance of its structure. He could see that structure and function were interdependent on every level, and he believed that in order for a system to increase its level of order and coherence, it must have sufficient energy to do so. Likewise, a lack of energy would decrease order, organisation, and would promote entropy in that system. This can be applied to the human body, and we see that the research supports this. The disease-state seems to be chaotic, where information transfer is poor and the system is incoherent as a whole, probably lacking in information. This is not limited to biological processes however, ala Gabor Mate and such individuals who show that the emotional/psychological state has the ability to promote or to cure disease. So in this sense, looking at the body almost like an information system which can be affected by all factors, external and internal, is probably the most constructive way to look at it. However, this thread is aimed at fixing some biological processes, so on this topic, I think we should attempt to increase the energy available to cell. We can do this by targeting the mitochondria, and by examining factors which are associated with defective mitochondrial metabolism.
Enter stress-metabolism
Any stressful stimuli (both external and physiological) requires increased metabolic efficiency to provide extra energy so that certain requirements can be met. If the stress persists, glycogen stores are eventually depleted (which happens fairly quickly), so adrenaline and cortisol are released via the HPA axis. Cortisol begins to break down muscle tissue, the liver performs gluconeogenesis and the proteins are converted to glucose. Adrenaline simultaneously activates lipolysis, breaking down adipose tissue and cell membranes fats to be used for energy by the mitochondria. However, due to the Randle Effect, fatty acids and glucose cannot undergo oxidative phosphorylation at the same time. Fatty acid oxidation inhibits pyruvate dehydrogenase (remember, the enzyme which allows pyruvate to enter the mitochondria), therefore glucose must undergo glycolysis. The simultaneous breakdown of fat via oxidative phosphorylation and glucose via glycolysis provides the body with maximum energy. However, the activation of HPA-axis hormones suppress the thyroid, and this process is only designed to be a temporary measure. The over production of lactate has several negative consequences, including further inhibition of mitochondrial respiration, and overall damage to cellular structures. A reliance on cortisol means that cholesterol's conversion to protective hormones pregnenelone, DHEA, and progesterone is stopped.
Ray Peat explains some of the processes involved in cancer "stress-metabolism" in the following:
Lactate formation from glucose is increased when anything interferes with respiratory energy production, but lactate, through a variety of mechanisms, can itself suppress cellular respiration. (This has been called the Crabtree effect.) Lactate can also inhibit its own formation, slowing glycolysis. In the healthy cell, the mitochondrion keeps glycolysis working by consuming pyruvate and electrons (or "hydrogens") from NADH, keeping the cell highly oxidized, with a ratio of NAD+/NADH of about 200. When the mitochondrion's ability to consume pyruvate and NADH is limited, the pyruvate itself accepts the hydrogen from NADH, forming lactic acid and NAD+ in the process. As long as lactate leaves the cell as fast as it forms, glycolysis will provide ATP to allow the cell to survive. Oxygen and pyruvate are normally "electron sinks," regenerating the NAD+ needed to produce energy from glucose.
But if too much lactate is present, slowing glycolytic production of ATP, the cell with defective respiration will die unless an alternative electron sink is available. The synthesis of fatty acids is such a sink, if electrons (hydrogens) can be transferred from NADH to NADP+, forming NADPH, which is the reducing substance required for turning carbohydrates and pyruvate and amino acids into fats.
This transfer can be activated by the transhydrogenase enzymes in the mitochondria, and also by interactions of some dehydrogenase enzymes.
The enzyme, fatty acid synthase (FAS), normally active in the liver and fat cells and in the estrogen-stimulated uterus, is highly active in cancers, and its activity is an inverse indicator of prognosis. Inhibiting it can cause cancer cells to die, so the pharmaceutical industry is looking for drugs that can safely inhibit it. This enzyme is closely associated with the rate of cell proliferation, and its activity is increased by both cortisol and estrogen.
The first biochemical event when a cell responds to estrogen is the synthesis of fat. Estrogen can activate transhydrogenases, and early studies of estrogen's biological effects provided considerable evidence that its actions were the result of the steroid molecule's direct participation in hydrogen transfers, oxidations and reductions. E.V. Jensen's claim that estrogen acts only through a "receptor protein" which activated gene transcription was based on his experimental evidence indicating that estrogen doesn't participate in oxidation and reduction processes in the uterus, but subsequently his claim has turned out to be false.
Glycolysis is very inefficient for producing usable energy compared to the respiratory metabolism of the mitochondria, and when lactate is carried to the liver, its conversion to glucose adds to the energy drain on the organism.
The hypoglycemia and related events resulting from accelerated glycolysis provide a stimulus for increased activity of the adaptive hormones, including cortisol. Cortisol helps to maintain blood sugar by increasing the conversion of protein to amino acids, and mobilizing free fatty acids from fat stores. The free fatty acids inhibit the use of glucose, so the stress metabolism relies largely on the consumption of amino acids. This increases the formation of ammonia, yet the combination of glycolysis and fat oxidation provides less carbon dioxide, which is needed for the conversion of ammonia to urea. Ammonia stimulates the formation of lactate, while carbon dioxide inhibits it.
Starving an animal with a tumor increases the stress hormones, providing free fatty acids and amino acids, and accelerates the tumor's growth (Sauer and Dauchy, 1987); it's impossible to "starve a tumor," by the methods often used. Preventing the excessive breakdown of protein and reducing the release of fatty acids from fat cells would probably cause many cancer cells to die, despite the availability of glucose, because of lactate's toxic effects, combined with the energy deficit caused by the respiratory defect that causes their aerobic glycolysis. Recently, the intrinsically high rate of cell death in tumors has been recognized. The tumor is maintained and enlarged by the recruitment of "stem cells." These cells normally would repair or regenerate the tissue, but under the existing metabolic conditions, they fail to differentiate properly.
The extracellular matrix in the tumor is abnormal, as well as the metabolites and signal substances being produced there, and the new cells fail to receive the instructions needed to restore the normal functions to the damaged tissue. These abnormal conditions can cause abnormal differentiation, and this cellular state is likely to involve chemical modification of proteins, including remodeling of the chromosomes through acetylation of the histones (Alam, et al., 2008; Suuronen, et al., 2006). The protein-protective effects of carbon dioxide are replaced by the protein-damaging effects of lactate and its metabolites.
The ability of lactic acid to displace carbon dioxide is probably involved in its effects on the blood clotting system. It contributes to disseminated intravascular coagulation and consumption coagulopathy, and increases the tendency of red cells to aggregate, forming "blood sludge," and makes red cells more rigid, increasing the viscosity of blood and impairing circulation in the small vessels. (Schmid-Schönbein, 1981; Kobayashi, et al., 2001; Martin, et al., 2002; Yamazaki, et al., 2006.)
The features of the stress metabolism include increases of stress hormones, lactate, ammonia, free fatty acids, and fat synthesis, and a decrease in carbon dioxide. Factors that lower the stress hormones, increase carbon dioxide, and help to lower the circulating free fatty acids, lactate, and ammonia, include vitamin B1 (to increase CO2 and reduce lactate), niacinamide (to reduce free fatty acids), sugar (to reduce cortisol, adrenaline, and free fatty acids), salt (to lower adrenaline), thyroid hormone (to increase CO2). Vitamins D, K, B6 and biotin are also closely involved with carbon dioxide metabolism. Biotin deficiency can cause aerobic glycolysis with increased fat synthesis (Marshall, et al., 1976).
A protein deficiency, possibly by increasing cortisol, is likely to contribute to increased FAS and fat synthesis (Bannister, et al., 1983), but the dietary protein shouldn't provide an excess of tryptophan, because of tryptophan's role as serotonin precursor--serotonin increases inflammation and glycolysis (Koren-Schwartzer, et al., 1994).
Incidental stresses, such as strenuous exercise combined with fasting (e.g., running or working before eating breakfast) not only directly trigger the production of lactate and ammonia, they also are likely to increase the absorption of bacterial endotoxin from the intestine. Endotoxin is a ubiquitous and chronic stressor. It increases lactate and nitric oxide, poisoning mitochondrial respiration, precipitating the secretion of the adaptive stress hormones, which don't always fully repair the cellular damage.
Aspirin protects cells in many ways, interrupting excitotoxic processes by blocking nitric oxide and prostaglandins, and consequently it inhibits cell proliferation, and in some cases inhibits glycolysis, but the fact that it can inhibit FAS (Beynen, et al., 1982) is very important in understanding its role in cancer.
There are several specific signals produced by lactate that can promote growth and other features of cancer, and it happens that aspirin antagonizes those: HIF, NF-kappaB, the kinase cascades, cyclin D1, and heme oxygenase.
Lactate and inflammation promote each other in a vicious cycle (Kawauchi, et al., 2008).
The toxic mechanism of bacterial endotoxin (lipopolysaccharide) involves inappropriate stimulation (Wang and White, 1999) of cells, followed by inflammation and mitochondrial inhibition. The stimulation seems to be a direct "biophysical" action on cells, causing them to take up water (Minutoli, et al., 2008), which is especially interesting, since estrogen's immediate excitatory effect causes cells to take up water.
The whole story is really complex, and there are so many avenues that link in with one another. I am only beginning to get my head around some of Peat's work, but I digress. A couple of things are for certain: Hypoxia (lack of oxygen) is present in almost every pathology, and in those that are not hypoxic, a defect in respiratory enzymes or complexes can be identified.
A small part of the picture: Nitric Oxide
Inefficient mitochondrial metabolism results in less production of carbon dioxide. Carbon dioxide is required by cells for several functional reasons, including the vasodilation of blood vessels, and maybe most importantly to allow the body to absorb oxygen via the respiratory tract. The amount of oxygen which can be absorbed from air is dependent on how much carbon dioxide is present in the blood, and this is due to the "Bohr Effect". Basically, if the mitochondria are not working properly, there will be low carbon dioxide (CO2), which also means that less oxygen diffuse into the blood stream. This is an initial cause of hypoxia. Remember that mitochondria need oxygen to produce ATP, so any oxygen deficiency is going to force many mitochondria to revert back to primitive glycolytic metabolism.
Additionally, since CO2 is the main vasodilator of blood vessels, in conditions of low CO2 the body is required upregulate the synthesis of Nitric Oxide (another potent vasodilator) via Nitric Oxide Synthase enzymes so that oxygen can reach the tissues. Nitric oxide elevations are meant to be a temporary measure against hypoxia.
However, during its own detoxification Nitric Oxide can bind "irreversibly" with Cytochrome C Oxidase, a key component of the mitochondrial electron transport chain. This means that for the cell to continue producing ATP via oxidative phosphorylation, it must produce a new cytochrome c oxidase, although this can be a lengthy and energy hungry process.
Regulation of mitochondrial respiration by nitric oxide inhibition of cytochrome c oxidase.
Nitric oxide (NO) and its derivatives inhibit mitochondrial respiration by a variety of means. Nanomolar concentrations of NO immediately, specifically and reversibly inhibit cytochrome oxidase in competition with oxygen, in isolated cytochrome oxidase, mitochondria, nerve terminals, cultured cells and tissues. Higher concentrations of NO and its derivatives (peroxynitrite, nitrogen dioxide or nitrosothiols) can cause irreversible inhibition of the respiratory chain, uncoupling, permeability transition, and/or cell death. Isolated mitochondria, cultured cells, isolated tissues and animals in vivo display respiratory inhibition by endogenously produced NO from constitutive isoforms of NO synthase (NOS), which may be largely mediated by NO inhibition of cytochrome oxidase. Cultured cells expressing the inducible isoform of NOS (iNOS) can acutely and reversibly inhibit their own cellular respiration and that of co-incubated cells due to NO inhibition of cytochrome oxidase, but after longer-term incubation result in irreversible inhibition of cellular respiration due to NO or its derivatives. Thus the NO inhibition of cytochrome oxidase may be involved in the physiological and/or pathological regulation of respiration rate, and its affinity for oxygen.
https://www.ncbi.nlm.nih.gov/pubmed/11239484
More on the destructive effects of excess Nitric Oxide:
The nitric oxide theory of aging revisited.
Bacterial and viral products, such as bacterial lipopolysaccharide (LPS), cause inducible (i) NO synthase (NOS) synthesis, which in turn produces massive amounts of nitric oxide (NO). NO, by inactivating enzymes and leading to cell death, is toxic not only to invading viruses and bacteria, but also to host cells. Injection of LPS induces interleukin (IL)-1beta, IL-1alpha, and iNOS synthesis in the anterior pituitary and pineal glands, meninges, and choroid plexus, regions outside the blood-brain barrier. Thereafter, this induction occurs in the hypothalamic regions (such as the temperature-regulating centers), paraventricular nucleus (releasing and inhibiting hormone neurons), and the arcuate nucleus (a region containing these neurons and axons bound for the median eminence). Aging of the anterior pituitary and pineal with resultant decreased secretion of pituitary hormones and the pineal hormone melatonin, respectively, may be caused by NO. The induction of iNOS in the temperature-regulating centers by infections may cause the decreased febrile response in the aged by loss of thermosensitive neurons. NO may play a role in the progression of Alzheimer's disease and parkinsonism. LPS similarly activates cytokine and iNOS production in the cardiovascular system leading to coronary heart disease. Fat is a major source of NO stimulated by leptin. As fat stores increase, leptin and NO release increases in parallel in a circadian rhythm with maxima at night. NO could be responsible for increased coronary heart disease as obesity supervenes. Antioxidants, such as melatonin, vitamin C, and vitamin E, probably play important roles in reducing or eliminating the oxidant damage produced by NO.
https://www.ncbi.nlm.nih.gov/pubmed/16399888
This suggests to me that Nitric Oxide in any excess is likely to have a destructive effect on health, especially if the mitochondria are already working suboptimally.
Methylene Blue: A potential solution for restoring mitochondrial efficiency
Heres a chemical which has some pretty amazing properties, and can be used in many cases to increase mitochondrial function, and in some cases actually reverse pathology.
Methylene blue (or MB) is a basic aniline dye with the molecular formula C16H18N3SCl. At room temperature, it appears as a solid, odorless, dark green powder that yields a blue solution when dissolved in water. It has many uses in a number of different fields. For instance, chemists use it to detect oxidizing agents and biologists use it to stain tissue samples and detect nucleic acids. In medicine, it is used as a treatment for various illnesses and disorders, including methemoglobinemia, schizophrenia, kidney stones, and herpes infections. In aquaculture, it is used to prevent freshwater fish eggs from being infected by bacteria and fungi.
Methylene blue has been described as "the first fully synthetic drug used in medicine." Methylene blue was first prepared in 1876 by German chemist Heinrich Caro.[40]
Its use in the treatment of malaria was pioneered by Paul Guttmann and Paul Ehrlich in 1891. During this period before the first World War, researchers like Ehrlich believed that drugs and dyes worked in the same way, by preferentially staining pathogens and possibly harming them. Methylene blue continued to be used in the second World War, where it was not well liked by soldiers, who observed, "Even at the loo, we see, we pee, navy blue." Antimalarial use of the drug has recently been revived.[41] It was discovered to be an antidote to carbon monoxide poisoning and cyanide poisoning in 1933 by Matilda Brooks.[42]
Among its many other benefits... I think some of the most interesting ones relate the it's effects on mitochondrial respiration:
1. Methylene Blue can artificially replace oxygen in the electron chain transport, meaning that hypoxia can essentially be mitigated and ATP synthesis can restored.
2. Methylene Blue can dissassociate Nitric Oxide from cytochrome C oxidase. From what I understand, the only other thing capable of doing this is red light.
3. Methylene Blue also inhibits Nitric Oxide Synthase
NOTE: It is best used in conjunction with red light, due to complementary physiological effects.
Here is an article from SelfHacked outlining some of the research on Methylene Blue:
1) Methylene Blue is an Anti-Depressant
Methylene Blue (MB) is a monoamine oxidase inhibitor (MAOI) (R).
MB inhibits MAO-A more than MAO-B, but it inhibits both at large doses (R).
At doses exceeding 5 mg/kg, it may cause serious serotonin toxicity/serotonin syndrome, if combined with any SSRIs or other serotonin reuptake inhibitor (R).
2)Methylene Blue is Anti-Cancer
Methylene blue appears to induce selective cancer cell apoptosis by the NQO1-dependent generation of cellular oxidative stress (R).
3) Methylene Can Help Alzheimer’s, Dementia and Parkinson’s and Huntington’s
Methylene blue has been investigated for the treatment of Alzheimer’s dementia (R, R2).
Methylene blue is proposed to affect neurodegeneration in Alzheimer’s disease via inhibition of tau protein aggregation and amyloids (R, R2).
It also may help Alzheimer’s by increasing acetylcholine (via acetylcholinesterase inhibition) (R).
It also partially repairs impairments in mitochondrial function and cellular metabolism (R).
By acting as an electron carrier and mitochondrial enhancer, it has promise in treating Parkinson’s disease (R).
Methylene blue helps Huntington’s by increasing autophagy and activating AMPK (R).
Methylene blue can alleviate mitochondrial abnormalities in a cellular model of progeria (R).
4) Methylene Blue Increases Blood Pressure
In diseased states, blood pressure often drops too low.
Methylene blue, an inhibitor of nitric oxide synthase and guanylate cyclase has been found to improve the hypotension associated with various clinical states (R).
5) Methylene Blue Improves Cognitive Performance in Health People
http://journal.frontiersin.org/article/10.3389/fphar.2015.00206/full
A randomized, double-blinded, placebo-controlled clinical trial of twenty-six subjects (age range, 22–62 years) was conducted on low dose methylene blue (~280mg, which is actually a high dose in my book) (R).
In this randomized study, low-dose methylene blue increased functional MR imaging activity during sustained attention and short-term memory tasks and potentiated memory retrieval (R).
Compared with control subjects, oral administration of low-dose methylene blue increased functional MR imaging response during the encoding, maintenance, and retrieval components of a short-term memory task in multiple clusters in the prefrontal, parietal, and occipital cortex (R).
Administration of methylene blue increased response (more brain activity) in the bilateral insular cortex during a psychomotor vigilance task (Z = 2.9–3.4, P= .01–.008) and functional MR imaging response during a short-term memory task involving the prefrontal, parietal, and occipital cortex (Z = 2.9–4.2, P = .03–.0003). Methylene blue was also associated with a 7% increase in correct responses during memory retrieval (P = .01) (R).
The insular cortex is important for sustained attention (R).
The study concluded: “Low-dose methylene blue can increase functional MR imaging activity during sustained attention and short-term memory tasks and enhance memory retrieval” (R).
Methylene blue has been shown to support memory consolidation and is neuroprotective as well (R).
In rat hippocampal slices, glutamate-mediated synaptic transmission is abolished by relatively high concentrations (5–50 mM) of MB (R).
On the other hand, MB is known to enhance memory retention and other brain functions in which ionotropic glutamate receptors are involved. It is possible that MB benefits cognitive function by modulating AMPA/kainate and NMDA-type ionotropic glutamate receptors (R).
Part of the cognitive enhancing effects are mediated by improvements in mitochondrial function.
6) Methylene Blue Helps Mitochondrial Function
It also has been shown that in low doses methylene blue protects the brain from disease by acting as an antioxidant in the mitochondria. It also acts as an artificial electron donor to complex I-IV of the mitochondria.
MB increases heme synthesis, cytochrome c oxidase (complex IV), and mitochondrial respiration (R), all of which help cognitive function.
This means that it can increase ATP production. ATP is the currency of life and the energy that powers humans. If our production of ATP declines, our physical and mental performance declines. Even healthy individuals can benefit from a boost in ATP production.
High concentrations of MB promote oxidative stress. Therefore, it is expected that low MB doses or concentrations will be, in general, more effective than large ones at facilitating physiological effects within mitochondria.
In fact, at high local concentrations, MB can potentially “steal” electrons away from the electron transport chain complexes, disrupting the redox balance and acting as a pro-oxidant (R).
It is well established that reduced MB can donate electrons to coenzyme Q and possibly to cytochrome c, thus increasing cytochrome oxidase (complex IV) activity and oxygen consumption (R).
At low concentrations, MB can interact with oxygen to form water, which would decrease the superoxide radicals produced during the process of oxidative phosphorylation. MB can also trap leaking electrons produced by mitochondrial inhibitors and preserve the metabolic rate by bypassing blocked points of electron flow, thus improving mitochondrial respiration (R, R2, R3).
In a rat model of cerebral ischemia, MB was able to speed up the removal of damaged mitochondria from a cell prior to cell death (mitophagy) (R).
MB is capable of reducing the mitochondrial damaging effects of amyloid beta in animal models (R).
Thus, MB is a potential target for mitochondrial dysfunction (R).
MB is able to stimulate glucose metabolism in conditions without oxygen (R), and increase NAD+ by mitochondria (R).
Some of the outcomes of improved mitochondrial function include increased fat burning (βeta-oxidation) (R), glucose utilization, ATP synthesis and extracellular matrix production (R).
In rat models of pancreatitis, Methylene blue reduces mitochondrial dysfunction (R).
7) Methylene Blue is Antimicrobial
Methylene blue was first used in 1891 to treat malaria.
Photodynamic therapy using the light-activated antimicrobial agent, MB kills methicillin-resistant staphylococcus aureus (MRSA) in superficial and deep excisional wounds (R).
MB in combination with light also inactivates viral nucleic acid of hepatitis-C and human immunodeficiency virus (HIV-1) and treats cases of resistant plaque psoriasis (R).
MB is an antifungal agent and can inhibit candida by causing mitochondrial dysfunction in this species (R).
8) Methylene Blue Can Extinguish Fear
Preclinical studies have shown that low-dose methylene blue increases memory retention after learning tasks, including fear extinction (R).
Adult participants displaying marked claustrophobic fear were randomly assigned to double-blind administration of 260 mg of methylene blue (N=23) or administration of placebo (N=19) immediately following six 5-minute extinction trials in an enclosed chamber (R).
The study concluded that Methylene blue enhances memory and the retention of fear extinction when administered after a successful exposure session but may have a deleterious effect on extinction when administered after an unsuccessful exposure session (R).
From: Methylene Blue: Revisited
METHYLENE BLUE IN SEPTIC SHOCK
A release of nitric oxide has been incriminated in the cardiovascular alterations of septic shock. Since guanylate cyclase is the target enzyme in the endothelium dependent relaxation mediated by nitric oxide, Methylene blue- a potent inhibitor of guanylate cyclase has been found very effective in improving the arterial pressure and cardiac function in septic shock.3
Studies have found improvement in mean arterial pressure (MAP) and systemic vascular resistance (SVR) while decreasing vasopressor requirements in septic shock.14
METHYLENE BLUE AND HEPATOPULMONARY SYNDROME
The hypoxemia in hepatopulmonary syndrome results from widespread pulmonary vasodilatation due to increased C-GMP. Methylene blue is found to ↑PaO2 and ↓alveolar-arterial difference for partial pressure of oxygen in all pts with hepatopulmonary syndrome. This was due to ↓C-GMP levels by Methylene Blue-a potent inhibitor of guanylate cyclase.2
METHYLENE BLUE AS ANTIMALARIAL
Methylene Blue has already been used some 100 yr ago against malaria, but it disappeared when chloroquine (CQ) and other drugs entered the market. However recent studies has shown the efficacy of Methylene Blue as an effective and cheap antimalarial agent especially in countries with increasing resistance of P. falciparum to existing 1st line antimalarial agents-CQ and pyrimethamine-sulfadoxine.
Methylene Blue, a specific inhibitor of P.falciparum glutathione reductase has the potential to reverse CQ resistance and it prevents the polymerization of haem into haemozoin similar to 4-amino-quinoline antimalarials.
A dose of 36-72mg/kg over 3 days is the most effective schedule.15
Apart from the intrinsic antimalarial activity and CQ sensitizing action it was also considered to prevent methemoglobinemia- a serious complication of malarial anemia.16
METHYLENE BLUE IN METHEMOGLOBINEMIA
Methemoglobinemia is a life threatening condition that can be congenital or acquired. It is characterized by the inability of hemoglobin to carry oxygen because the ferrous part of the heme molecule has been oxidized to a ferric state.
Methylene Blue acts by reacting within RBC to form leukomethylene blue, which is a reducing agent of oxidized hemoglobin converting the ferric ion (fe+++) back to its oxygen carrying ferrous state(fe++).17
Dose commonly used is 1-2mg/kg of 1% Methylene Blue solution.17,18
METHYLENE BLUE AND CANCER
Recent research suggests that Methylene Blue and other redox cyclers induce selective cancer cell apoptosis by NAD (P) H: quinine oxidoreductase (NQO1)-dependent bioreductive generation of cellular oxidative stress. Hence Methylene Blue is being investigated for the photodynamic treatment of cancer.19
IFOSFAMIDE NEUROTOXICITY
Another, less well known use of Methylene Blue is its utility for treating ifosfamide neurotoxicity. A toxic metabolite of ifosfamide, chloroacetaldehyde, disrupts the mitochondrial respiratory chain, leading to accumulation of nicotinamide adenine dinucleotide hydrogen (NADH).
Methylene blue acts as an alternative electron acceptor, and reverses the NADH inhibition of hepatic gluconeogenesis while also inhibiting the transformation of chloroethylamine into Chloroacetaldehyde, and also inhibits multiple amine oxidase activities, preventing the formation of Chloroacetaldehyde.20
Hence it has prophylactic and therapeutic role in ifosfamide - induced encephalopathy.21
NEUTRALIZATION OF HEPARIN
Methylene blue effectively neutralizes heparin especially in pts with protamine allergy. However work still needs to be done to determine the safety of the drug at the higher doses necessary to neutralize heparin levels achieved in bypass patients.26
METHYLENE BLUE AND PRIAPISM
Methylene blue has been used to treat high flow priapism by intra-cavernous injection which is known to antagonize endothelial derived relaxation factor.27
METHYLENE BLUE AND ALZHEIMER'S DISEASE
The relationship between Methylene blue and Alzheimer's disease has recently attracted increasing scientific attention. It has been shown to attenuate the formations of amyloid plaques and neurofibrillary tangles and partial repair of impairments in mitochondrial function and cellular metabolism.28
METHYLENE BLUE COMBINED WITH LIGHT
Photodynamic therapy using the light activated anti-microbial agent, Methylene blue kills methicillin resistant staphylococcus aureus (MRSA) in superficial and deep excisional wounds.29 Methylene blue in combination with light also inactivates viral nucleic acid of hepatitis-C and human immunodeficiency virus (HIV-1) and treats cases of resistant plaque psoriasis.30,31
https://www.ncbi.nlm.nih.gov/pmc/articles/PMC3087269/
Methylene blue-mediated photodynamic therapy enhances apoptosis in lung cancer cells
Combined treatment with a photosensitizer and iodide laser [photodynamic therapy (PDT)] has improved the outcome of various cancers. In this study, we investigated the effects of using the photosensitizer methylene blue (MB) in PDT in human lung adenocarcinoma cells. We found that MB enhances PDT-induced apoptosis in association with downregulation of anti-apoptotic proteins, reduced mitochondrial membrane potential (MMP), increased phosphorylation of the mitogen-activated protein kinase (MAPK) and the generation of reactive oxygen species (ROS). In MB-PDT-treated A549 cells, we observed PARP cleavage, procaspase-3 activation, downregulation of the anti-apoptotic proteins Bcl-2 and Mcl-1, and the reduction of mitochondrial membrane potential (MMP). Western blot data showed that phosphorylation of p38 was increased in MB-PDT-treated A549 cells, indicating that several signaling molecules participate in the apoptotic cascade. Our data also showed that apoptotic cell death in MB-PDT-treated cells occurred through a series of steps beginning with the photochemical generation of ROS. Demonstrating the role of ROS, pretreatment of A549 cells with the antioxidant N-acetylcysteine (NAC) followed by MB-PDT resulted in increased cell viability and reduced proteolytic cleavage of PARP.
https://www.spandidos-publications.com/10.3892/or.2013.2494
Photodynamic therapy with intralesional methylene blue and a 635 nm light-emitting diode lamp in hidradenitis suppurativa: a retrospective follow-up study in 7 patients and a review of the literature
Hidradenitis suppurativa is a chronic inflammatory skin disease which has an estimated prevalence of 1%. It is characterized by the formation of recurrent painful suppurative nodules and abscesses in the flexural areas of the body. It is believed that its pathogenesis involves an aberrant, genetically-determined activation of innate immunity against the bacterial commensal flora of intertriginous areas. It has been found that the formation of antibiotic-resistant bacterial biofilms is a common finding in hidradenitis lesions. Photodynamic therapy with different compounds and light sources has demonstrated its efficacy in a number of infectious diseases such as nail mycosis and chronic periodontitis. We retrospectively report our experience in the treatment of hidradenitis with photodynamic therapy using intralesional methylene blue and a 635 nm light-emitting diode lamp in 7 patients. Two patients received one session whereas 5 patients received two sessions. At one month follow-up good response was achieved in 6 patients. After 6 months, 5 patients (71%) maintained remission of the disease in the treated area. In view of the results and literature review, we regard methylene blue as an ideal photosensitizer for photodynamic therapy in this disease.
http://pubs.rsc.org/en/Content/ArticleLanding/2016/PP/c6pp00082g#!divAbstract
Methylene blue alleviates experimental autoimmune encephalomyelitis by modulating AMPK/SIRT1 signaling pathway and Th17/Treg immune response.
Methylene blue (MB) is an effective neuroprotectant in many neurological disorders. AMP-activated protein kinase (AMPK)/silent mating-type information regulation 2 homolog 1 (SIRT1) plays a crucial role in maintaining inflammatory responses and shows a synergistic effect on cell homeostasis. We investigated the effect of MB on experimental autoimmune encephalomyelitis (EAE), a classical animal model of multiple sclerosis (MS). MB treatment reduced the clinical scores of EAE significantly and attenuated pathological injuries in spinal cords. Furthermore, the protective effects of MB were related to the activation of AMPK/SIRT1 signaling pathway. In addition, MB treatment alleviated T helper type17 (Th17) responses and increased regulatory T cell (Treg) responses. Our findings suggest that MB could be a promising reagent to treat autoimmune diseases and MS